I still need to post about the pros and cons of nuclear energy. However, this post is my explanation for why I've not posted anything for a week.
How do internal combustion engines work? This is not a question posed to me by my son, my friends, or anyone else. I'll explain the purpose of posing this at the end.
An internal combustion engine like what you have in your vehicle works by mixing fuel (gasoline, diesel, etc.) with air, compressing it to very high pressures, (or compressing the fuel to high pressures and then mixing with air in the case of diesel), and then causing the mixture to explode or at least expand quickly, usually via some sort of spark (gasoline, etc.) or other high temperature device (glow plug: diesel). This explosion pushes a piston to move down through the combustion cylinder. That moving piston turns something called a crank-shaft. This crank-shaft is connected to some sort of transmission. That transmission transmits (duh!) the rotational energy from the engine into rotational energy in the wheels via a drive train (which includes a bunch of stuff). There's a flywheel attached to the crank-shaft, which causes the crank-shaft to want to continue turning, even after the piston has reached its lowest point. The crank-shaft is designed such that at the piston's lowest point, it will push the piston back up through the cylinder, starting the internal combustion process anew. This happens over and over and over, causing two to four thousand revolutions of the crank-shaft per minute.
All of these metal parts moving against each other cause tremendous amounts of heat to be generated. The exploding fuel mixture transfers a lot of the waste heat to the engine components. So, there's a system for lubricating and one for cooling an internal combustion engine.
First, the moving parts are immersed in a low viscosity fluid that resists degradation at high temperatures. This is the engine oil. Engine oil is designed as a lubricant that can be heated to high temperatures without changing its properties very much. This lubricant has one main job: to keep the hot metal parts moving smoothly against each other.
The second part to keeping the engine working is the cooling system. There are two parts to this cooling system. First, the engine block is cast (usually in aluminum, steel, or sometimes ceramic) with a network of piping between the outer wall and the cylinders through which a cooling fluid flows. Secondly, a pump pushes that fluid through the block and into a radiator. The radiator has many small pipes which the fluid moves through. This gives the fluid a high surface area, allowing it to quickly cool as relatively cold air passes through the radiator. This works via a mechanism called forced convection (the name "radiator" is a misnomer). That cooled fluid is then pumped back into the engine block and is heated as it moves through the block back to the radiator. The coolant moves around and around, transporting heat from the engine and losing that heat to the surrounding air via the radiator. This effectively and efficiently keeps the engine at a reliable temperature so all of those moving parts can work well.
Here's the thing. The correct, reliable operation of the engine relies on efficient, well-calibrated cooling. That cooling requires coolant. When your radiator develops a hole through which the coolant can escape, the engine can no longer be cooled. When that occurs, a number of nasty things happen:
1) The engine and all of its parts heat up more than they're supposed to.
2) The oil, which is specially formulated to work under specific conditions is no longer working under those conditions, so it starts to degrade.
3) If the oil degrades significantly, it no longer lubricates the moving metal parts, allowing them to scrape against each other.
4) If the oil degrades significantly, it may vaporize, greatly increasing the pressure inside the engine. That pressure increase can be so great that it blows out the gaskets (seals) in the engine block. This is what is meant when you hear someone say they "blew out a head gasket."
5) If the pressure and temperature is a bit higher, the head (the part that the spark plugs and valves and some other components connect to, just above the pistons) can be warped due to the quickly changing size (materials change size when they change temperature) being mitigated in some places by bolts.
6) If the pressure is really high, it can crack the engine block.
I don't know how bad it is yet, but I almost certainly blew out the head gasket on my brand new 11000 mile, 2007 Tacoma. I may have cracked the block, and being the pessimist that I am, I suspect that is the case. Even if I didn't crack the block, the pistons may have scored the cylinders, in which case I might as well have cracked the block (the cylinders in this engine are specially lined and cannot be repaired once they're scored).
Toyota's MSRP for a brand new engine is $15,000. A rebuilt engine runs around $3-4k. If I only blew out the head gasket or warped the head, I can repair it myself for the cost of parts and several weeks to months of working on it in the evenings. If the block is cracked, I need a "new" engine. If the cylinders are scored, I need a "new" engine.
If I need a new engine: I'm toying with the idea of replacing the V6 with an 8-cylinder diesel engine modified to run on biodiesel, veggie oil, or other renewable fuels. But, I need to address some other issues before I can even seriously consider going that route (such as how certain things like stability control and other safety issues are specifically handled and whether they require the gasoline engine to operate correctly). There wouldn't be much difference in cost between a "new" gasoline engine and a "new" diesel engine.
Unfortunately, the truck is sitting in the street by our house because it completely stopped running just as I got home. November 1 is "snow plow" day, meaning we can no longer park on the street.
I cannot/will not start taking apart the engine to assess the damage in the street. I ordered a new radiator from Toyota on Tuesday, but when I went to pick it up today, I discovered they had ordered the one for a manual transmission. The automatic transmission in my truck relies on the radiator to help keep IT at the correct operating temperature. That's another story.
Anyway, I can't move the truck right now because I can't start it without a radiator, which I won't get until Monday. I can't assess the damage because it's not in my garage, and I can't even see how much oil is/was in the cooling system because the hose doesn't reach from the house to the truck (I just bought a new hose this evening while I was out getting the radiator that isn't the radiator), so I can't flush out what's currently in the block to see how bad the damage is.
I HAVE to move the truck before Saturday evening. I happen to have an anchor bolt built into the floor of my garage. I do not know how much stress it can handle, but it's pretty beefy. I plan on attaching one end of a come-a-long to the anchor and the other end to the truck and pulling it up the steep driveway, into the garage. The house happens to be at the top of a hill with a straight shot down the street to a rather cross street. Perhaps you'll see me on the 9 O'clock news this weekend. Don't worry, I'll be careful.
So, I've been rather bummed and not too excited about writing about nuclear energy. My apologies. I'll get back to it this weekend or early next week.
Thursday, October 30, 2008
Wednesday, October 22, 2008
Nuclear energy: How it works
I promised to talk about how nuclear electricity generation works, its pros and cons, and whether I think it should be a part of our future energy portfolio.
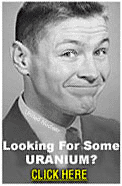
(Kerry loves this little nerd, so asked for it to be included in my post.)
First, I need to say a bit about how atoms (specifically, their nuclei) are put together. This little page has a great primer on nuclear energy in general, and I'll be cribbing from it liberally.
Nuclei are made up of protons and neutrons. These are both relatively massive particles in the atomic world, but the mass of a nucleus is always less than the sum of the individual masses of the protons and neutrons which constitute it. The difference is a measure of the nuclear binding energy which holds the nucleus together. This binding energy can be calculated from the Einstein relationship, which you've seen before. E=mc^2. Only this time, the "m" is a difference in mass between the sum of the individual masses of the protons and neutrons and the actual mass of the nucleus, and E is the energy that holds those neutrons and protons together.
Here is a plot of the binding energy vs nuclide mass or number of nucleons in the atomic nucleus. Nickel 62 is the most tightly bound nuclide. You'll often see it claimed, incorrectly, that Fe56 is the most tightly bound nuclide. This comes from astrophysics where Fe56 is the most common stable nuclide produced in stellar nucleosynthesis processes.

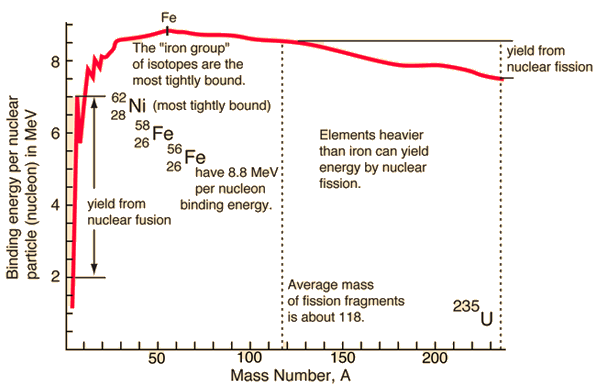

So, as you can see in the plot, you can release the nuclear binding energy by one of two processes. Fission is the process in which you break apart a nucleus of a heavy element (Uranium, for example), creating lighter elements and energy, with some possible other particles released as well. Fusion is the process in which you push two light nuclides together until they form a new element, releasing a bunch of energy. Fusion happens in stars, generating helium and heavier elements from hydrogen. Fission happens in nuclear bombs and nuclear power plants. Fission also happens naturally. We cannot yet get more energy out of a fusion event than we put in, so I will only speak about fission. Interestingly, we can get quite a bit more energy from fusion than from fission, but we need incredible amounts of energy to control the reactions; once we can figure out how to cheaply create the environment at the center of the sun, we can have much, much, much more than enough cheap and "easy" energy... Oh, wait, we have such an environment only 1 AU from us... ;)
Nuclear fission can happen with elements heavier than those in the "iron group", which includes Fe56 and Ni62. The very basic idea is that a slow moving neutron is collided with a massive nuclide (say, Uranium 235). During this collision, Uranium 235 absorbs the nuclide and its energy. Suddenly the nucleus has more energy than the binding energy required to hold it together. This means that there's enough energy to break the nucleus apart. The products are, in one instance, Rb90 and Cs143, a few neutrons, and a bunch of energy. Rb90 and Cs143 are unstable, with half-lives on the order of minutes to seconds; we'll talk about that next time.
Notice that the mass of Rb90+Cs143 is not 235. That missing mass is mostly in the form of energy (about 200 million times as much energy as the slow moving neutron had). There are a few more neutrons as well, but not enough to account for the missing mass. We'll talk about those neutrons in a bit, but first we have to explain "slow moving" neutron.
We need a slow moving neutron because a fast moving neutron won't be captured. In order to control the speed of the neutron, we use something called a neutron moderator. The most common moderator is light water (doesn't have deuterium). That's what is meant by a "light water reactor". There are a number of other moderators in use, and several being researched. These moderators produce what we call "thermal" or "slow moving" neutrons from faster neutrons.
Back to those newly produced neutrons from the neutron+U235 collision. We can use those neutrons to collide with other U235 nuclei, giving us a chain reaction. If we can control this reaction by only allowing one (no more, no less) neutron from each collision to go on to another collision, we can have a sustained but controlled nuclear fission reaction. We control the number of neutrons allowed to participate in collisions by means of the control rods, usually made of boron or cadmium. These elements can absorb many neutrons without themselves becoming unstable. The control rods could get too hot, which would cause them to melt and stop being effective in controlling the chain reaction, causing something like the Three Mile Island accident (like, not identical to).
If we have fewer than one neutron going on to collide with more uranium, the reaction dies and everything stops. No energy generation. If we allow more than one neutron to collide with more uranium, we have an uncontrolled chain reaction with a positive feedback loop, very quickly releasing a lot more energy than we could possibly control in the reactor. This is basically what nuclear bombs do, but it's a lot more complicated, depending on the way things happen.
To maintain the temperature of the control rods, the core, and other components of the reactor, water is used as a coolant. The energy released is so much that the water is quickly converted to steam. This steam is used to drive turbines. The steam-driven turbines generate the electricity, much like coal- or natural gas-fired plants steam-driven turbines.
This has been a very simple and basic description of the process by which nuclear power is used to generate electricity. I'm going to have to add another post or two to discuss the pros and cons and other elements to nuclear energy.
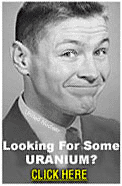
(Kerry loves this little nerd, so asked for it to be included in my post.)
First, I need to say a bit about how atoms (specifically, their nuclei) are put together. This little page has a great primer on nuclear energy in general, and I'll be cribbing from it liberally.
Nuclei are made up of protons and neutrons. These are both relatively massive particles in the atomic world, but the mass of a nucleus is always less than the sum of the individual masses of the protons and neutrons which constitute it. The difference is a measure of the nuclear binding energy which holds the nucleus together. This binding energy can be calculated from the Einstein relationship, which you've seen before. E=mc^2. Only this time, the "m" is a difference in mass between the sum of the individual masses of the protons and neutrons and the actual mass of the nucleus, and E is the energy that holds those neutrons and protons together.
Here is a plot of the binding energy vs nuclide mass or number of nucleons in the atomic nucleus. Nickel 62 is the most tightly bound nuclide. You'll often see it claimed, incorrectly, that Fe56 is the most tightly bound nuclide. This comes from astrophysics where Fe56 is the most common stable nuclide produced in stellar nucleosynthesis processes.

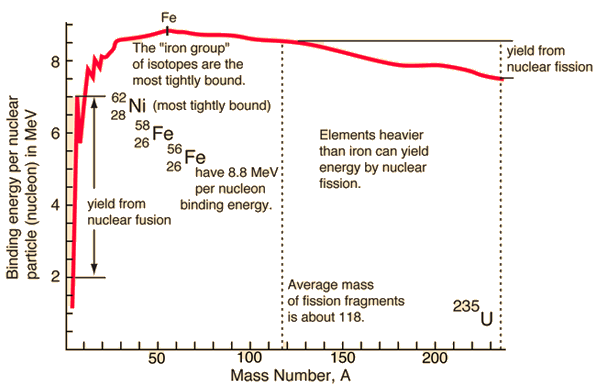

So, as you can see in the plot, you can release the nuclear binding energy by one of two processes. Fission is the process in which you break apart a nucleus of a heavy element (Uranium, for example), creating lighter elements and energy, with some possible other particles released as well. Fusion is the process in which you push two light nuclides together until they form a new element, releasing a bunch of energy. Fusion happens in stars, generating helium and heavier elements from hydrogen. Fission happens in nuclear bombs and nuclear power plants. Fission also happens naturally. We cannot yet get more energy out of a fusion event than we put in, so I will only speak about fission. Interestingly, we can get quite a bit more energy from fusion than from fission, but we need incredible amounts of energy to control the reactions; once we can figure out how to cheaply create the environment at the center of the sun, we can have much, much, much more than enough cheap and "easy" energy... Oh, wait, we have such an environment only 1 AU from us... ;)
Nuclear fission can happen with elements heavier than those in the "iron group", which includes Fe56 and Ni62. The very basic idea is that a slow moving neutron is collided with a massive nuclide (say, Uranium 235). During this collision, Uranium 235 absorbs the nuclide and its energy. Suddenly the nucleus has more energy than the binding energy required to hold it together. This means that there's enough energy to break the nucleus apart. The products are, in one instance, Rb90 and Cs143, a few neutrons, and a bunch of energy. Rb90 and Cs143 are unstable, with half-lives on the order of minutes to seconds; we'll talk about that next time.
Notice that the mass of Rb90+Cs143 is not 235. That missing mass is mostly in the form of energy (about 200 million times as much energy as the slow moving neutron had). There are a few more neutrons as well, but not enough to account for the missing mass. We'll talk about those neutrons in a bit, but first we have to explain "slow moving" neutron.
We need a slow moving neutron because a fast moving neutron won't be captured. In order to control the speed of the neutron, we use something called a neutron moderator. The most common moderator is light water (doesn't have deuterium). That's what is meant by a "light water reactor". There are a number of other moderators in use, and several being researched. These moderators produce what we call "thermal" or "slow moving" neutrons from faster neutrons.
Back to those newly produced neutrons from the neutron+U235 collision. We can use those neutrons to collide with other U235 nuclei, giving us a chain reaction. If we can control this reaction by only allowing one (no more, no less) neutron from each collision to go on to another collision, we can have a sustained but controlled nuclear fission reaction. We control the number of neutrons allowed to participate in collisions by means of the control rods, usually made of boron or cadmium. These elements can absorb many neutrons without themselves becoming unstable. The control rods could get too hot, which would cause them to melt and stop being effective in controlling the chain reaction, causing something like the Three Mile Island accident (like, not identical to).
If we have fewer than one neutron going on to collide with more uranium, the reaction dies and everything stops. No energy generation. If we allow more than one neutron to collide with more uranium, we have an uncontrolled chain reaction with a positive feedback loop, very quickly releasing a lot more energy than we could possibly control in the reactor. This is basically what nuclear bombs do, but it's a lot more complicated, depending on the way things happen.
To maintain the temperature of the control rods, the core, and other components of the reactor, water is used as a coolant. The energy released is so much that the water is quickly converted to steam. This steam is used to drive turbines. The steam-driven turbines generate the electricity, much like coal- or natural gas-fired plants steam-driven turbines.
This has been a very simple and basic description of the process by which nuclear power is used to generate electricity. I'm going to have to add another post or two to discuss the pros and cons and other elements to nuclear energy.
Saturday, October 18, 2008
So, my friend Jennifer asked the following about my previous post about nuclear energy:
The first question is easier. I'm an advocate of reducing pollution, greenhouse gases, and reducing how much of the aeons-old materials we pull from the ground. As was made clear in my first post, basically everything we use comes from the ground. In my opinion, we should take as little mass from the ground as possible. How do we do that?
It's the old mantra: Reduce, Reuse, Recycle. This can be accomplished in many ways, and there are many blogs out there about what specific people do. My bestest friend in the whole wide world (and also my wife) has a bunch of blogs she follows that discuss all different aspects of doing just that.
So, if we reduce the amount of material we're using, we'll very quickly notice that a lot of the energy generation we "needed" is also reduced. We'll notice that the atmospheric pollution we generate is greatly reduced. If we also reduce the amount of energy we directly use (drive less, turn off lights, use more efficient lights and appliances, etc.), we'll notice another, greater drop in pollution.
So, first, I think we should just stop using as much (of energy and stuff) as we use now.
Now, there is some amount of energy we'll need to continue generating to maintain our "standard of living." We can get a lot of that energy from solar, wind, hydrothermal, and hydroelectric. I dare say that we could generate ALL of our energy needs from those four sources. Let me back that up:
There was a math error in the paragraph below. I've corrected it, and had to reduce the fudge factor to a factor of two instead of ten. The point is still the same, it's just not as wildly liberal with how easy it would be to replace all US electricity generation with solar. Corrections are in purple while bad values are in red.
Solar panels are about 12% efficient right now. That means that a cell that covers 0.01 m^2 generates about 1.2 Watts on a clear day. The US uses about 3.2x10^12 watts per year. That means we would need about 2.7*10^12 solar cells that cover 0.01 m^2 each to generate all of our power needs. That's 2.7x10^9 m^2. This is incorrect; the correct value is 2.7*10^10 m^2. That's 27000 km^2. The surface area of the US is 9.2 million km^2 of land. Arizona has about 300,000 km^2. So, let's add 50% of the solar panel surface area to account for the infrastructure. The following statement is a little too liberal due to the math error above. This is a wild guess--we could even double or increase by a factor of 10---it doesn't really matter. Naw, let's use a factor of 10 more, to account for infrastructure, more panels because of the fact that not 100% of the Arizona days are clear, etc. Instead, let's increase by a factor of two to account for infrastructure, etc. So, 54000 km^2 is needed for solar panels to generate 100% of the United State's energy needs. That's about 1/5 of Arizona's land. That's a lot. However, we have a lot of land in the southwest: New Mexico, California, etc. We also have a LOT of roofs in the southwest. We could easily provide more energy than the entire US needs with solar panels alone, at today's efficiencies.
It would be extremely difficult to get all of that energy across the US using the current power grid. Let's add some wind generators in the midwest, some hydrothermal in areas like Yellowstone and Alaska, maybe some wave (I don't like wave much, mostly because I don't know enough about it) on the coasts and Hawaii, etc. We can, with current technology and just a little bit of will, completely stop using coal- and natural-gas fired power plants in a decade or less if we had real leaders in technology and politics, and it wouldn't require the use of ANY nuclear plants.
My next post will directly address nuclear, because I don't think we should necessarily take it off of the table; 27,000 km^2 of solar panels is a LOT of mining for silicon.
So am I seeing here that you are an advocate of nuclear energy assuming we were not going to put more effort into solar and wind power?Warning: This is going to be rather opinionated, ranting, and preachy. :)
I'd be interested in hearing more about the nuclear process and the pros/cons of it as a reliable and safe energy source.
The first question is easier. I'm an advocate of reducing pollution, greenhouse gases, and reducing how much of the aeons-old materials we pull from the ground. As was made clear in my first post, basically everything we use comes from the ground. In my opinion, we should take as little mass from the ground as possible. How do we do that?
It's the old mantra: Reduce, Reuse, Recycle. This can be accomplished in many ways, and there are many blogs out there about what specific people do. My bestest friend in the whole wide world (and also my wife) has a bunch of blogs she follows that discuss all different aspects of doing just that.
So, if we reduce the amount of material we're using, we'll very quickly notice that a lot of the energy generation we "needed" is also reduced. We'll notice that the atmospheric pollution we generate is greatly reduced. If we also reduce the amount of energy we directly use (drive less, turn off lights, use more efficient lights and appliances, etc.), we'll notice another, greater drop in pollution.
So, first, I think we should just stop using as much (of energy and stuff) as we use now.
Now, there is some amount of energy we'll need to continue generating to maintain our "standard of living." We can get a lot of that energy from solar, wind, hydrothermal, and hydroelectric. I dare say that we could generate ALL of our energy needs from those four sources. Let me back that up:
There was a math error in the paragraph below. I've corrected it, and had to reduce the fudge factor to a factor of two instead of ten. The point is still the same, it's just not as wildly liberal with how easy it would be to replace all US electricity generation with solar. Corrections are in purple while bad values are in red.
Solar panels are about 12% efficient right now. That means that a cell that covers 0.01 m^2 generates about 1.2 Watts on a clear day. The US uses about 3.2x10^12 watts per year. That means we would need about 2.7*10^12 solar cells that cover 0.01 m^2 each to generate all of our power needs. That's 2.7x10^9 m^2. This is incorrect; the correct value is 2.7*10^10 m^2. That's 27000 km^2. The surface area of the US is 9.2 million km^2 of land. Arizona has about 300,000 km^2. So, let's add 50% of the solar panel surface area to account for the infrastructure. The following statement is a little too liberal due to the math error above. This is a wild guess--we could even double or increase by a factor of 10---it doesn't really matter. Naw, let's use a factor of 10 more, to account for infrastructure, more panels because of the fact that not 100% of the Arizona days are clear, etc. Instead, let's increase by a factor of two to account for infrastructure, etc. So, 54000 km^2 is needed for solar panels to generate 100% of the United State's energy needs. That's about 1/5 of Arizona's land. That's a lot. However, we have a lot of land in the southwest: New Mexico, California, etc. We also have a LOT of roofs in the southwest. We could easily provide more energy than the entire US needs with solar panels alone, at today's efficiencies.
It would be extremely difficult to get all of that energy across the US using the current power grid. Let's add some wind generators in the midwest, some hydrothermal in areas like Yellowstone and Alaska, maybe some wave (I don't like wave much, mostly because I don't know enough about it) on the coasts and Hawaii, etc. We can, with current technology and just a little bit of will, completely stop using coal- and natural-gas fired power plants in a decade or less if we had real leaders in technology and politics, and it wouldn't require the use of ANY nuclear plants.
My next post will directly address nuclear, because I don't think we should necessarily take it off of the table; 27,000 km^2 of solar panels is a LOT of mining for silicon.
Friday, October 17, 2008
Question from my dad
So, my dad asked the following question the other night.
"If we have 1 kilogram of water, what is the maximum amount of energy we could collect from it, assuming Einstein is right and the equation E=mc^2 is correct?"
First, we have to assume some things that are not exactly correct.
1) We could actually "collect" that 'E' above in some fashion.
2) We can turn 100% of the energy into useful work.
So, E=mc^2:
in words:
Kinetic energy is given by the mass of an object times its velocity (we saw this when talking about the small asteroid impacting in Sudan a topic back). Let's assume that we can actually recover 100% of the energy of 1 kilogram of water moving at the speed of light. Ignore the fact that we cannot accelerate a massive particle to the speed of light. The reality of the equation above is that the kinetic energy of 1 kilogram of water (anything, in truth) moving at the speed of light is given by the above equation, not that we can get that much energy from a kilogram of something moving at that speed.
The speed of light is approximately 3x10^8 m/s.
So, the energy involved in 1 kilogram of something moving at the speed of light:
E = 1*(3x10^8)^2 = 9*10^16 Joules (that basically the same as 1x10^17 joules).
Okay. Let's assume we can convert all of that (impossible) kinetic energy into usable energy, say electricity. How long could we power the United States?
According to wikipedia, the US uses 105 exajoules in one year (2005). That's 10^20 joules. So, 1 kilogram of water would provide 1/1000 of the energy used in the US in one year, assuming you could capture and use 100% of the kinetic energy of 1 kilogram moving at the speed of light (ignore that the Lorentz equations require inifinite energy to accelerate anything with mass TO the speed of light).
How much energy do we get from oil? 4*10^19 joules, or about 40% of our total usage. So, if we could capture the energy of 400-500 kg moving at the speed of light and turn 100% of it into energy, we could cease using any oil and continue to power the US at its current consumption rate.
Let's get a little more realistic now.
How much energy can we get from 1 kg of fuel in a nuclear reactor? According to this site, we can get about 1.7x10^11 joules of usable energy in a year. That's about 1 billion times less energy than the US uses. We're currently getting about 8% of our energy from nuclear. That implies that we're using about .08*10^9 = 80,000,000 kg of nuclear fuel today.
However, wikipedia indicates that a large reactor can generate about 10^15 joules from 1 kg of fuel. So, I have an error bar of four orders of magnitude on the energy density of nuclear fuels. That means, if these two sources are at all reliable, we're using something between 8 thousand and 80 million kg of nuclear fuel to provide us with 8% of our energy.
Obviously, this is much better than the mass used in the chemical energy generation (fossil fuel burning). 1 kg of coal will produce about 5.2*10^7 joules of energy. We use about 2.5*10^19 joules of coal-produced energy in a year, so we're using something on the order of 4*10^11 kg of coal a year. We get about 6.2*10^7 joules of energy from one kg of oil. Since we use about 4*10^19 joules of energy (per year) from oil, we're using about 6.5*10^11 kg of oil per year. We use about 2.5*10^19 joules of gasoline per year. We get about 2.6*10^7 joules per kg of gasoline, so we're using about 9.6^11 kg of gasoline per year.
In total, we're using 9.6*10^11 + 4*10^11 + 6.5*10^11 kg = 2*10^12 kg of fossil fuels per year. Compared with up to 8*10^7 kg of nuclear fuel per year.
Even if we decided to go 100% nuclear, with rounding up, we'd be using at most 100 times less material to go fully nuclear. Remember, we're mining both materials out of the ground. Of course, there are issues with making nuclear safe, but there are as many pressing issues with making fossil fuels safe.
[preaching]
The WHO estimates 2.4 million people die each year due to pollution. Let's (unfairly, maybe) lump all of that pollution into that generated by the burning of fossil fuels. Deaths due to nuclear plant operation? About 1000 per year, including all of the disasters and the very slight increases in cancer risk to the public (did you know that coal-fired plants release more radioactive materials than nuclear plants do?).
Here's a nice chart that shows the normalized deaths due to electricity generation:
Now, add the deaths due to pollution from burning fossil fuels and nuclear power plant-caused deaths are way, way, way below the noise. That's ignoring the increased safety of newer nuclear plants, and that chart doesn't have the deaths due to pollution from burning coal. If you include the deaths due to pollution, that normalized deaths increases to something like 8000 for coal and natural gas.
Imagine we could stop using coal and natural gas and replace them with nuclear, and assume we use the higher number of kg quoted above, just to give us the increase in material we'd need as a proxy for the increase in number of plants we'd need. Assuming a linear increase in the number of normalized deaths with number of plants, we'd have about 800 deaths per TWy for nuclear. Zero for coal+natural gas. That's a net decrease (assuming 8,000 deaths due to coal, natural gas, and pollution) of about 7000 deaths per TWy. The world uses about 16 TW per year. That would mean about 112,000 fewer deaths per year. Considering most of those deaths are due to pollution, we'd also be raising the quality of life of many, many people.
Why isn't the decrease about 2.4 million if all of the deaths due to pollution are due to coal- and natural gas-fired power plants? Because we burn gasoline too, and wood for heating, etc. Also, the above approximations are very poor and linear usually doesn't work well. Given all of that, it's safe to say that a 100% decrease in the use of fossil fuel-fired power plants would make life a lot better for a lot of people, even if we add in the risks due to nuclear fuel.
Of course, if we used less energy, generated a higher % of it from things like solar, created less trash, etc., we'd be even better off.
[/preaching]
"If we have 1 kilogram of water, what is the maximum amount of energy we could collect from it, assuming Einstein is right and the equation E=mc^2 is correct?"
First, we have to assume some things that are not exactly correct.
1) We could actually "collect" that 'E' above in some fashion.
2) We can turn 100% of the energy into useful work.
So, E=mc^2:
in words:
Kinetic energy is given by the mass of an object times its velocity (we saw this when talking about the small asteroid impacting in Sudan a topic back). Let's assume that we can actually recover 100% of the energy of 1 kilogram of water moving at the speed of light. Ignore the fact that we cannot accelerate a massive particle to the speed of light. The reality of the equation above is that the kinetic energy of 1 kilogram of water (anything, in truth) moving at the speed of light is given by the above equation, not that we can get that much energy from a kilogram of something moving at that speed.
The speed of light is approximately 3x10^8 m/s.
So, the energy involved in 1 kilogram of something moving at the speed of light:
E = 1*(3x10^8)^2 = 9*10^16 Joules (that basically the same as 1x10^17 joules).
Okay. Let's assume we can convert all of that (impossible) kinetic energy into usable energy, say electricity. How long could we power the United States?
According to wikipedia, the US uses 105 exajoules in one year (2005). That's 10^20 joules. So, 1 kilogram of water would provide 1/1000 of the energy used in the US in one year, assuming you could capture and use 100% of the kinetic energy of 1 kilogram moving at the speed of light (ignore that the Lorentz equations require inifinite energy to accelerate anything with mass TO the speed of light).
How much energy do we get from oil? 4*10^19 joules, or about 40% of our total usage. So, if we could capture the energy of 400-500 kg moving at the speed of light and turn 100% of it into energy, we could cease using any oil and continue to power the US at its current consumption rate.
Let's get a little more realistic now.
How much energy can we get from 1 kg of fuel in a nuclear reactor? According to this site, we can get about 1.7x10^11 joules of usable energy in a year. That's about 1 billion times less energy than the US uses. We're currently getting about 8% of our energy from nuclear. That implies that we're using about .08*10^9 = 80,000,000 kg of nuclear fuel today.
However, wikipedia indicates that a large reactor can generate about 10^15 joules from 1 kg of fuel. So, I have an error bar of four orders of magnitude on the energy density of nuclear fuels. That means, if these two sources are at all reliable, we're using something between 8 thousand and 80 million kg of nuclear fuel to provide us with 8% of our energy.
Obviously, this is much better than the mass used in the chemical energy generation (fossil fuel burning). 1 kg of coal will produce about 5.2*10^7 joules of energy. We use about 2.5*10^19 joules of coal-produced energy in a year, so we're using something on the order of 4*10^11 kg of coal a year. We get about 6.2*10^7 joules of energy from one kg of oil. Since we use about 4*10^19 joules of energy (per year) from oil, we're using about 6.5*10^11 kg of oil per year. We use about 2.5*10^19 joules of gasoline per year. We get about 2.6*10^7 joules per kg of gasoline, so we're using about 9.6^11 kg of gasoline per year.
In total, we're using 9.6*10^11 + 4*10^11 + 6.5*10^11 kg = 2*10^12 kg of fossil fuels per year. Compared with up to 8*10^7 kg of nuclear fuel per year.
Even if we decided to go 100% nuclear, with rounding up, we'd be using at most 100 times less material to go fully nuclear. Remember, we're mining both materials out of the ground. Of course, there are issues with making nuclear safe, but there are as many pressing issues with making fossil fuels safe.
[preaching]
The WHO estimates 2.4 million people die each year due to pollution. Let's (unfairly, maybe) lump all of that pollution into that generated by the burning of fossil fuels. Deaths due to nuclear plant operation? About 1000 per year, including all of the disasters and the very slight increases in cancer risk to the public (did you know that coal-fired plants release more radioactive materials than nuclear plants do?).
Here's a nice chart that shows the normalized deaths due to electricity generation:
Fuel | Immediate fatalities 1970-92 | Who? | Normalised to deaths per TWy* electricity |
Coal | 6400 | workers | 342 |
---|---|---|---|
Natural gas | 1200 | workers & public | 85 |
Hydro | 4000 | public | 883 |
Nuclear | 31 | workers | 8 |
Now, add the deaths due to pollution from burning fossil fuels and nuclear power plant-caused deaths are way, way, way below the noise. That's ignoring the increased safety of newer nuclear plants, and that chart doesn't have the deaths due to pollution from burning coal. If you include the deaths due to pollution, that normalized deaths increases to something like 8000 for coal and natural gas.
Imagine we could stop using coal and natural gas and replace them with nuclear, and assume we use the higher number of kg quoted above, just to give us the increase in material we'd need as a proxy for the increase in number of plants we'd need. Assuming a linear increase in the number of normalized deaths with number of plants, we'd have about 800 deaths per TWy for nuclear. Zero for coal+natural gas. That's a net decrease (assuming 8,000 deaths due to coal, natural gas, and pollution) of about 7000 deaths per TWy. The world uses about 16 TW per year. That would mean about 112,000 fewer deaths per year. Considering most of those deaths are due to pollution, we'd also be raising the quality of life of many, many people.
Why isn't the decrease about 2.4 million if all of the deaths due to pollution are due to coal- and natural gas-fired power plants? Because we burn gasoline too, and wood for heating, etc. Also, the above approximations are very poor and linear usually doesn't work well. Given all of that, it's safe to say that a 100% decrease in the use of fossil fuel-fired power plants would make life a lot better for a lot of people, even if we add in the risks due to nuclear fuel.
Of course, if we used less energy, generated a higher % of it from things like solar, created less trash, etc., we'd be even better off.
[/preaching]
Thursday, October 9, 2008
Why is 0 times everything 0?
Why is 0 times everything 0?
To answer this question, I first have to give you the field axioms and equation logic:
Equation logic:
Reflexivity: s = s
Symmetry: if s=t, t=s.
Transitivity: if s=t and t=v, s=v.
Substitution: if s=t and v is a member of the field, s*v = t*v.
Substitution: if s=t and v is a member of the field, s+v = t+v.
There's another rule of logic that's irrelevant to what we're doing here.
These axioms and logic rules define everything you can do with numbers in the various fields (complex numbers, real numbers and rational numbers, for example. The set of integers is not a field because you cannot mathematically invert any given integer and get an integer back.
Okay, let's use the above axioms and equation logic to prove that, for any number A that is part of a field, A*0=0.
1: 0=0 (reflexivity)
2: 0+0=0 (additive identity)
3: A*(0+0) = A*0 (logical substitution; multiplication is well-defined).
4: A*0 + A*0 = A*0 (multiplicative distribution)
5: A*0 + A*0 + -A*0 = A*0 + -A*0 (logical substitution; addition is well-defined)
6: A*0 + (A + -A)*0 = 0*(A + -A) (multiplicative distribution)
7: A*0 + 0*0 = 0*0 (additive inverse)
8: let 0*0 = b. (simplification, and therefore:)
9: A*0 + b = b
10: A*0 + b + -b = b + -b (logical substitution; addition is well-defined)
11: A*0 + 0 = 0 (additive inverse)
12: A*0 = 0 (additive identity)
13: QED (pompousness)
There you have it. For any number A in a field that obeys the above axioms and rules of logic, A*0 = 0.
To answer this question, I first have to give you the field axioms and equation logic:
name | addition | multiplication | ||
associativity | ![]() | ![]() | ||
commutativity | ![]() | ![]() | ||
distributivity | ![]() | ![]() | ||
identity | ![]() | ![]() | ||
inverses | ![]() | ![]() |
Equation logic:
Reflexivity: s = s
Symmetry: if s=t, t=s.
Transitivity: if s=t and t=v, s=v.
Substitution: if s=t and v is a member of the field, s*v = t*v.
Substitution: if s=t and v is a member of the field, s+v = t+v.
There's another rule of logic that's irrelevant to what we're doing here.
These axioms and logic rules define everything you can do with numbers in the various fields (complex numbers, real numbers and rational numbers, for example. The set of integers is not a field because you cannot mathematically invert any given integer and get an integer back.
Okay, let's use the above axioms and equation logic to prove that, for any number A that is part of a field, A*0=0.
1: 0=0 (reflexivity)
2: 0+0=0 (additive identity)
3: A*(0+0) = A*0 (logical substitution; multiplication is well-defined).
4: A*0 + A*0 = A*0 (multiplicative distribution)
5: A*0 + A*0 + -A*0 = A*0 + -A*0 (logical substitution; addition is well-defined)
6: A*0 + (A + -A)*0 = 0*(A + -A) (multiplicative distribution)
7: A*0 + 0*0 = 0*0 (additive inverse)
8: let 0*0 = b. (simplification, and therefore:)
9: A*0 + b = b
10: A*0 + b + -b = b + -b (logical substitution; addition is well-defined)
11: A*0 + 0 = 0 (additive inverse)
12: A*0 = 0 (additive identity)
13: QED (pompousness)
There you have it. For any number A in a field that obeys the above axioms and rules of logic, A*0 = 0.
Tuesday, October 7, 2008
And we have impact.
Well, the 2-3 meter diameter rock that was predicted to hit in/near Sudan has (also here). The atmospheric impact was detected with what's called an infrasound detector. An infrasound detector detects shock wave (sound) events in the low frequency range (0.02 to 20 hz) and can be used to make all kinds of cool measurements. One of the things that can be derived from the observations is the energy of the event.
The Sudan atmospheric impact's energy release was between 1.1 and 2.1 kilotons of TNT. That's about 1012 calories or 4.184 terajoules. Little Boy released between 13 and 18 kilotons of TNT equivalent energy. Tunguska, another atmospheric asteroid explosion released about 5 to 30 megatons of TNT equivalent energy. These little rocks are moving so fast that they could really do some damage...
[rant]If you think NASA's budget is too high, consider the above information carefully. We can now detect rocks just a few meters in diameter. Such small rocks release 1/10 of the energy of the atomic bomb that destroyed Hiroshima. A rock just a little more massive, faster or bigger is a pretty scary proposition. An asteroid a lot bigger or more massive is quite nasty and we need to better understand our solar system so we can better understand our place in it. [/rant]
We can, with some basic assumptions, work out the density of this asteroid, knowing nothing more than the amount of energy released, the size, and the escape velocity of Earth. I assume this information is somewhere out there, but let's just do this for fun. ;)
We all know that the kinetic energy of a body is its velocity squared times its mass. An object impacting the earth from space is moving close to the earth's escape velocity.
E=mv^2.
E = 4.184x10^12 J
v=11.86 km/s = 11860 m/s
(the actual velocity was 12.8 km/s, so let's use that instead of the escape velocity of the Earth)
m = E/v^2
m = (4.184x10^12 kg m^2/s^2)/(12800m/s)^2
= 4.184x10^12 kg m^2/s^2)/(163840000 m^2/s^2)
= 25,537 kg
Assume a spherical rock 3 m in diameter. Its volume is 4/3 * Pi * r^3 = 4/3 * Pi * 1.5^3 = about 14 m^3.
The density of an object is mass divided by volume. 25537/14 =1824 kg/m^3. That's the density of a rubble pile.
This asteroid was not made of nickel/iron. Nor was it solid rock; this was rock with lots of pore space (gaps between the rocks).
Let's go back to my little rant about more massive rocks. Let's assume this rock, with the same density, was ten times larger in diameter (not too unrealistic).
Its volume would be: 14000 m^3. With a density of 1800 kg/m^3, its mass would be 25,200,000 kg. If it were moving at the same velocity of 12800 m/s, the total energy released would be:
E=mv^2
E = 25,200,000 kg * (12800 m/s)^2
E = 25,200,000 kg * (163840000 m^2/s^2)
E = 4.12 * 10^15 joules.
That's about a megaton of TNT. That's 100 times as powerful as the bomb that destroyed Hiroshima.
The Sudan atmospheric impact's energy release was between 1.1 and 2.1 kilotons of TNT. That's about 1012 calories or 4.184 terajoules. Little Boy released between 13 and 18 kilotons of TNT equivalent energy. Tunguska, another atmospheric asteroid explosion released about 5 to 30 megatons of TNT equivalent energy. These little rocks are moving so fast that they could really do some damage...
[rant]If you think NASA's budget is too high, consider the above information carefully. We can now detect rocks just a few meters in diameter. Such small rocks release 1/10 of the energy of the atomic bomb that destroyed Hiroshima. A rock just a little more massive, faster or bigger is a pretty scary proposition. An asteroid a lot bigger or more massive is quite nasty and we need to better understand our solar system so we can better understand our place in it.
We can, with some basic assumptions, work out the density of this asteroid, knowing nothing more than the amount of energy released, the size, and the escape velocity of Earth. I assume this information is somewhere out there, but let's just do this for fun. ;)
We all know that the kinetic energy of a body is its velocity squared times its mass. An object impacting the earth from space is moving close to the earth's escape velocity.
E=mv^2.
E = 4.184x10^12 J
v=11.86 km/s = 11860 m/s
(the actual velocity was 12.8 km/s, so let's use that instead of the escape velocity of the Earth)
m = E/v^2
m = (4.184x10^12 kg m^2/s^2)/(12800m/s)^2
= 4.184x10^12 kg m^2/s^2)/(163840000 m^2/s^2)
= 25,537 kg
Assume a spherical rock 3 m in diameter. Its volume is 4/3 * Pi * r^3 = 4/3 * Pi * 1.5^3 = about 14 m^3.
The density of an object is mass divided by volume. 25537/14 =1824 kg/m^3. That's the density of a rubble pile.
This asteroid was not made of nickel/iron. Nor was it solid rock; this was rock with lots of pore space (gaps between the rocks).
Let's go back to my little rant about more massive rocks. Let's assume this rock, with the same density, was ten times larger in diameter (not too unrealistic).
Its volume would be: 14000 m^3. With a density of 1800 kg/m^3, its mass would be 25,200,000 kg. If it were moving at the same velocity of 12800 m/s, the total energy released would be:
E=mv^2
E = 25,200,000 kg * (12800 m/s)^2
E = 25,200,000 kg * (163840000 m^2/s^2)
E = 4.12 * 10^15 joules.
That's about a megaton of TNT. That's 100 times as powerful as the bomb that destroyed Hiroshima.
Monday, October 6, 2008
Not a question...
Okay, this post is not a question from G. It's just frickin' cool.
This is an email forwarded to me from...well, someone else. ;)
Two meters! My size (in one dimension anyway)! That's the size of the object detected, tracked, and confirmed by independent observers, with 98%+ probability, to hit the Earth's atmosphere tonight (I believe it'll be Sudan's night). If you don't think this is awesome, wicked cool, and other sundry adjectives, I don't know what to tell you. :)
This is an email forwarded to me from...well, someone else. ;)
NEO News (10/06/08) Bolide impact predicted tonight!
This is a first: a very small asteroid (or rock) has been discovered that is on course for an impact tonight in Sudan. This information is from various reports to posted to MPML (the Minor Planet Mailing List at groups.yahoo.com/group/mpml/). The impactor is only about 2 m across and will break up in the atmosphere, with no risk to those on the ground. (If something this size hit in the daytime, it would probably not be noticed, but at night it should put on quite show).
Alan Harris writes that this object, with the survey-assigned designation 8TA9D69, was discovered by the University of Arizona Mt. Lemmon survey and will almost certainly, tonight, become the first impacting bolide discovered before entry into the Earth's atmosphere. Steve Chesley (JPL) reports that atmospheric entry will occur on 2008 Oct 07 0246 UTC over northern Sudan.
Andrea Milani of the University of Pisa wrote the following: Today the object with the provisional designation 8TA9D69 was submitted to impact monitoring by using the normal software of the NEODyS system, by using the observations as reported by the MPC on the NEO Confirmation Page. Based on 26 optical observations from 2008/10/06.278 to 2008/10/06, the probability of impact is between 99.8% and 100%; in practice the impact can be considered sure and is for tonight. Our computation has already been confirmed independently by others, including the JPL NEO Program Office (with which we consult in all relevant cases of possible impact). The effect of this atmospheric impact will be the release, in either a single shot or maybe a sequence of explosions, of about 1 kiloton of energy. This means that the damage on the ground is expected to be zero. The location of these explosions is not easy to predict due to the atmospheric braking effects. The only concern is that they might be interpreted as something else, that is man-made explosions. Thus in this case, the earlier the public worldwide is aware that this is a natural phenomenon, which involves no risk, the better.
This is the first time an asteroid impact has been predicted, and it reflects the increasing capability of the Spaceguard Survey. There was one previous false alarm when, for a few hours around Christmas 2004, it appeared that an impact by a 30-m asteroid was possible, but this was ruled out by additional observations. The current case, however, seems much more solid.
Two meters! My size (in one dimension anyway)! That's the size of the object detected, tracked, and confirmed by independent observers, with 98%+ probability, to hit the Earth's atmosphere tonight (I believe it'll be Sudan's night). If you don't think this is awesome, wicked cool, and other sundry adjectives, I don't know what to tell you. :)
Friday, October 3, 2008
How is fuel made?
How is fuel made? Gasoline for cars, specifically.
Oh, boy.
First, the crude oil is extracted from the ground. That's a different story...
Linky
The crude oil is brought to a refinery, where it is pumped through a high temperature steam boiler. This boiler heats the crude oil to about 600 to 700 degrees celsius. This superheated crude oil is pumped into a distillation tower.
The distillation tower has several levels of progressively lower temperatures, with the highest temperature at the bottom and the lowest at the top. Most of the components of the crude oil will vaporize at or below 600 C. Because of the temperature profile of the refining tower, certain chemicals will be condensed at certain heights. This difference in condensation temperature allows the separation of chemicals as their gaseous forms rise through the tower.
The stuff that won't vaporize at below 700 C or so is called the residual crude---tar, coke, some waxes, etc. These are solids or very refractory liquids that are often used in making other things.
At about 370 to 600 degrees C, heavy oil condenses. This is used as an industrial fuel or used to make other products.
At about 300 to 370 degrees C, lubricating oil (stuff used to lubricate your car engine), grease, etc.) condenses.
At about 250 to 350 degrees C, diesel fuel, heating oil, etc. condense. These are sometimes used to make other products.
At about 175 to 325 degrees C, kerosene condenses. Kerosene is used in jet fuel, tractor fuel, lantern oil, etc. Often used to make other products, too.
At about 40 to 205 degrees C, gasoline condenses. This is the fuel most of us use in our cars.
At about 60 to 100 degrees C, naptha condenses. Naptha is used to make high octane gasoline, as an industrial solvent, etc.
At about 40 degrees C, petroleum gas condenses. This is used to make LPG, to make plastics, etc.
Oh, boy.
First, the crude oil is extracted from the ground. That's a different story...
Linky
The crude oil is brought to a refinery, where it is pumped through a high temperature steam boiler. This boiler heats the crude oil to about 600 to 700 degrees celsius. This superheated crude oil is pumped into a distillation tower.
The distillation tower has several levels of progressively lower temperatures, with the highest temperature at the bottom and the lowest at the top. Most of the components of the crude oil will vaporize at or below 600 C. Because of the temperature profile of the refining tower, certain chemicals will be condensed at certain heights. This difference in condensation temperature allows the separation of chemicals as their gaseous forms rise through the tower.
The stuff that won't vaporize at below 700 C or so is called the residual crude---tar, coke, some waxes, etc. These are solids or very refractory liquids that are often used in making other things.
At about 370 to 600 degrees C, heavy oil condenses. This is used as an industrial fuel or used to make other products.
At about 300 to 370 degrees C, lubricating oil (stuff used to lubricate your car engine), grease, etc.) condenses.
At about 250 to 350 degrees C, diesel fuel, heating oil, etc. condense. These are sometimes used to make other products.
At about 175 to 325 degrees C, kerosene condenses. Kerosene is used in jet fuel, tractor fuel, lantern oil, etc. Often used to make other products, too.
At about 40 to 205 degrees C, gasoline condenses. This is the fuel most of us use in our cars.
At about 60 to 100 degrees C, naptha condenses. Naptha is used to make high octane gasoline, as an industrial solvent, etc.
At about 40 degrees C, petroleum gas condenses. This is used to make LPG, to make plastics, etc.
Subscribe to:
Posts (Atom)