Saturday, December 27, 2008
T6 Wedge fracture
The doctor's "education materials":
You have a compression fracture of one of the bones in your spine. This fracture occurs after minor injury (falling to the ground) in older persons with osteoporosis (thinning of the bones). It may also occur in young healthy persons after a severe trauma (car accident or fall from a height). This is a stable fracture and does not cause any injury to the spinal cord or nerves. This injury will take 4-6 weeks to heal and can be treated at home with bed rest and pain medicine.
In other words, a broken back. Linky, linky, linky...
This, my friends, is what happened to my DW this morning while sledding with our son.
There is this new, manufactured hill in town where the city has been doing construction to widen the roads, add a walk- and bike-path, and make it easier to cross town. We had driven past a few times and seen dozens of people out having fun. G has been asking if we could go. For Christmas, G received a sled on runners from his grandfather (GL); it was GL's when he was a boy. So, this morning, we decided to go and try out the hill with the "new" sled and a plastic sled we had purchased a week or so ago. We arrived and a big highway sign said, "No Parking, NOT a snowplay area." Yeah, right. Everyone was playing in the snow. Obviously this was a snowplay area.
So, we found a suitably steep slope, put G on the runners sled, and sent him off. He went all the way down, across the basin, and back up the other hill a bit. What fun! I came after him on the plastic sled. It was fast, and hard. I managed not to hurt myself. DW went next and she bounced along the slope. When we were all back at the top, DW said that we should find a more shallow slope. We did, G went down, and had fun. I went down, had fun. We came back up and went down again. G got tangled in his sled, hurt his knee a touch, but was fine. I went down on my stomache, without a sled. Slow, and fine. DW went next on the plastic sled, hit a jump (not purposely) at the bottom of the hill, and took flight. She landed on her back, compressing her T6 vertebra (not that we knew that at the time). A man nearby said he had probably broken a rib yesterday on this slope (but he was out again...). We went back up, G went down again, and then DW asked us to take her home. After some prodding by me, we went to the urgent care, and then to the ER (because UC doesn't do back x-rays). After a few hours at ER (very little wait in the lobby---we were triaged through pretty quickly), we got the above education.
The ER apparently receives about 22 sledding accident cases per day in winter. There were at least three sledding cases in there with us, one person was so bad off that he couldn't walk.
Apparently, the best way to go sledding is on your stomach, head first (with a bicycle helmet). Don't go on your bottom because you are very likely to get this kind of compression fracture in your back.
So, now DW is on hydrocodone, a mild, narcotic, semi-synthetic opiate. So, apparently she's a user. We're all keeping an eye on her. At least any cough she may have had will be relieved.
I don't have a scanner, so I can't show you her x-ray, but here are some good examples.
Be careful out there.
Next time, barring any other sudden cases of education, we'll be examining the potential dangers of nuclear energy w.r.t. accidents or sabotage.
Wednesday, December 24, 2008
Cons to nuclear power, part deux
3) Proliferation.
This basic argument against developing new nuclear energy technologies or power plants is based on the idea that somehow this nuclear technology can be used by rogues to develop nuclear weapons. The basic information on how to enrich uranium is already out there. I just discussed it in my previous posts. Gas diffusion is not difficult to accomplish. Centrifuge diffusion is not difficult to accomplish. Sure, it's expensive, but anyone who wants to and has the money can do it. So, why is the state department afraid of proliferation?
A) Nuclear enrichment abused; Iran as an example: Iran is a signatory of the Nuclear non-Proliferation Treaty (NPT), which basically says that only the P-5 (US, Russia, UK, France, and China), who have declared that they are nuclear weapons states, may produce or stockpile nuclear weapons. All signatories commit to collaborate on developing peaceful uses of nuclear energy. Iran is an NPT signatory. Russia has been helping Iran with nuclear fuel technologies, including enrichment. So far, that's all kosher according to the NPT... Enriching past the nuclear fuel to nuclear weapons grade uranium or plutonium basically just requires more diffusion cycles and more energy. Iran has more than enough oil and natural gas reserves to supply itself with energy for many many decades. Thus, the US is concerned that Iran is using the Russian technologies to build nuclear weapons rather than nuclear fuel. Considering Iran's wacked stance on some things, it's a valid concern, IMO.
Note that India and Pakistan, both of whom have had nuclear weapons since 1998 are not NPT signatories. North Korea is a signatory, as is South Africa.
So, we have several rogues who have or want to have nuclear weapons technologies. What does this have to do with nuclear power generation (specifically in the US)? Well, IMO, it has nothing to do with it. You cannot stop knowledge from proliferating. Just because Iran may be working toward having "The Bomb" doesn't mean we should necessarily hobble ourselves w.r.t. energy generation. Once a person or state has the knowledge of enrichment, they can go as far as they desire (and can afford) with that enrichment, so the only way we can stop them is to either destroy them or convince them it's not in their self-interest to build a bomb. The former isn't a reasoned option, so what can our state deptartment do to stop Iran from building a bomb? I dunno; I'm just a scientist, not a statesman, but I suspect our current path hasn't been working so well.
B) Redirection of already enriched nuclear fuel: This is theft or direct purchase of enriched fuel by a state or sub-state or group of wackos. Obviously the more states that have nuclear enrichment capabilities, the more opportunities to acquire enriched fuel that can then be made weapons-grade relatively easily. MIT estimates that there is enough enriched plutonium, produced from nuclear energy fuel generation, in Europe, Russia, and Japan to produce about 25,000 nuclear weapons, assuming 8kg/weapon. Obviously, only a few weapons are needed to greatly destabalize any given region.
Proliferation summary:
The means (knowledge and technology) of acquiring a nuclear weapon (via advanced enrichment) is not something we can easily control (see North Korea and Iran). The motive to acquire and use a nuclear weapon is something we (the US in particular) have not recently been very good at mitigating (see Iran). The opportunity to acquire a nuclear weapon or the fuel needed to make one is something we (the US) can stop within our borders and sphere of influence relatively easily. Stopping theft or purchase of Russian (for example) nuclear technology or fuel is not something we seem to have any hand in. The NPT has been fairly effective, but it only takes one state to feel threatened by another before it'll do what it feels it has to...
So, the expansion of today's nuclear power plants to non-nuclear (powered but not weaponed) states does pose some risks. Obviously the detonation of a nuclear weapon anywhere is cause for concern, and would likely be answered with a nuclear weapon. The cascade of responses could go all the way to WWIII. I do not know. I do not think it would take a nuclear weapon to start another world war, but I suspect the use of one would probably guarantee another large, if not world-wide war. This is obviously undesireable.
So, how can we mitigate the risks of nuclear weapons proliferation while still expanding nuclear power generation (or is it even possible)?
For one, the current fuel cycle is a pathway to weapons technology. There are other fuel cycles that do not lead to enriched, weapons-grade plutonium or uranium. These are not in wide-spread use, but they can be developed more aggressively and then spread to those countries that are looking to move to nuclear energy technologies.
For another, diffusing many of the tensions throughout the world is probably a good idea, regardless of the state of nuclear power proliferation. We do not need nuclear weapons to kill a lot of people...
Anyone who wants to can work out how to build a basic reactor. Such things are not difficult to do. The difficulty lies in ensuring that people don't want to move on to nuclear weapons...
I promised to say something about downblending in this topic's post. Downblending is the process of mixing weapons-grade and weapons-usable enriched fuels with depleted fuels to decrease the concentration of the fissible materials, in the process making nuclear power-grade fuels. This is a "new" way of more safely decreasing nuclear weapons stockpiles. In some ways this decreases proliferation risks by decreasing the ready availability of weapons-usable HEU that can be used in crude weapons, which can be made by relatively poor countries or groups (crude being similar in destructive scale to those used in WWII...).
Anyway, proliferation is a concern, but... The knowledge necessary for enriching materials to weapons-usable or weapons-grade form is easily obtainable, the technology isn't much more difficult to obtain, there is no need for nuclear weapons to kill many people or destabilize a region, and decreasing the desire to kill many people seems (to me) to be a more prudent approach than attempting to retroactively close Pandora's Box...
Saturday, December 20, 2008
Cons to nuclear power
Why should we NOT increase our use of nuclear power?
There are a number of arguments against nuclear power. The list below is not in any particular order.
1) cost
2) waste
3) proliferation
4) accidents or sabatoge
1) Cost: This is---at best---an inane argument, IMO. We can spend the money now on new technologies or we can spend the money later on health care, global warming mitigation, etc. Saying "it's too expensive to build or develop new nuclear power plants," is just whining. Yes, I am perfectly happy to be dismissive of and condescending to anyone arguing against nuclear power on the basis of cost.
2) Waste: This is the best argument (IMO, of course) against nuclear power.
2A) To get the uranium ore, we must mine for it. This creates long-lived contamination around the mining site. The uranium tailings contain over a dozen radioactive species, including thorium-230, radium-226, radon-222, and polonium-210. These are all hazardous materials, of course. If, as in past practices, the radioactive sand is left on the surface, it could be blown about by the wind, washed away by rains, etc. There is not actually a high concentration (compared with other nuclear waste materials) of hazardous materials by mass, but there will always be a large mass of tailings from any given mine. The most serious and probable human health hazard associated with the tailings is lung cancer caused by inhaling these radioactive products.
Anti-arguments: Here's the thing, though... The most dangerous, common rock, in terms of radioactivity, is granite. Your granite tabletop (if you're so lucky) is continuously producing radon gas. It's not much, and it's certainly not a health concern. Your drywall plaster is probably made of calcined gypsum. That's releasing more ionizing radiation than your granite counter top, and it's in every room of your house. It's still not a health concern. All the portland cement you see and use (home foundations, sidewalks, some freeways, buildings, etc., etc., etc.) are emitting as much or more radon as fly ash from coal-fired power plants. The uranium tailings from old mines are certainly dangerous, but new techniques in dealing with the tailings are in place, and even better ones are being developed. That's a pretty hand-wavy argument that the waste from mining is "safe." It's not safe. Neither is burning coal. Here is a paper that argues that the radiation dosage from mine tailings isn't terribly dangerous. That's only one side of the story, of course. This paper estimates the dosage someone living around a coal-fired plant will be exposed to. This paper discusses the comparison between coal and portland cement sources of radon. We see more cancers from pollution and cement-derived radon in our homes than we do from mine tailings, and increased nuclear power generation will only decrease the overall number of cancers, even if we continued to use the worst methods of dealing with uranium tailings.
2B) To get fissionable material (U235) from the uranium ore (uranium oxide, "yellow cake"), it must go through enrichment. (Stolen from here): Natural uranium is composed of 0.72% U-235 (the fissionable isotope), 99.27% U-238, and a trace quantity 0.0055% U-234 . The 0.72% U-235 is not sufficient to produce a self-sustaining critical chain reaction in U.S. style light-water reactors, although it is used in Canadian CANDU reactors. For light-water reactors, the fuel must be enriched to 2.5-3.5% U-235. Uranium is found as uranium oxide which when purified has a rich yellow color and is called "yellowcake". After reduction, the uranium must go through an isotope enrichment process. Even with the necessity of enrichment, it still takes only about 3 kg of natural uranium to supply the energy needs of one American for a year.
The byproducts of enrichment are basically depleted uranium (DU, which is pretty much just U238) and uranium hexafluoride gas (UF6). U238 is relatively safe, with a half-life of about 4.5 billion years, via the path of alpha decay. Alpha particles are just helium nuclei. They can be dangerous when ingested or inhaled, but are pretty much harmless externally (alpha particles are stopped by a single layer of dead skin cells). That's not to say that alpha decay is harmless. Radon gas, polonium 210, etc. are implicated in cancers and other nasty deaths if inhaled (Pu210 is thought to have been the material used to poison Alexander Litvinenko). U238 is also chemically toxic, and can have seriously bad effects on the liver, once again if ingested. U238 is now being used in a process called "downblending." I'll discuss downblending in the proliferation discussion. UF6 is a different beast altogether. UF6 is an extremely unstable waste compound that's not easy to store or handle. Here you can find a 118 page PDF on safe handling procedures for UF6. I haven't read it. Let it suffice to say that UF6 is mostly bad because of its chemical (not nuclear) toxicity. The uranium part of UF6 is obviously radioactive. The depleted UF6 (with mostly U238, not U235) is stored as waste. Any amount of water mixed with UF6 will turn it into a rather nasty acid, so the storage of UF6 is a difficult task.
I cannot argue against the dangers of depleted UF6... It is as dangerous as any other toxic chemical used in other manufacturing processes. Since it also contains U238, it's scary to a lot of people. I'm not any more concerned about UF6 than any other toxic chemical (melamine?) that is moved around this country and others.
2A and 2B are the wastes from the processing and creation of nuclear fuel. There are wastes that come out the back end of the nuclear power generation process.
2C) Spent fuel rods contain fisson products that emit beta and gamma particles. They also contain actinides that emit alpha particles. These products include uranium-234, neptunium-237, plutonium-238 and americium-241, and even sometimes some neutron emitters such as californium (Cf). None of these are pleasant products. Some are so radioactively hot that they're thermally hot.
Many countries, the US excluded, reprocess these products to extract any left-over U235 for re-use as a nuclear fuel. This increases the concentration of the radioactive products in the waste. It also increases the concentration of the toxic chemicals used to process the materials. The US just stores the waste as-is, without reprocessing. One can argue either way about what to do with these products. Since we're in the US, I'll briefly mention Yucca Mountain.
Some geologists have certified that Yucca Mountain would be a safe place to store these waste products. Others have argued that it is not safe. I'm not sure who is correct. I'm not sure how dangerous these products are in the long term. I am sure that if we were to spend some money paying scientists and engineers, they could figure out a workable solution. It would not be ideal, it would not please everyone.
So, waste is the biggie. It's not solved, but it's not nearly as bad as many people think.
I think this post is long enough for now. I'll post arguments 3 and 4 later...
Friday, November 14, 2008
Awesome!
First, though, I want to rave about the discoveries that are making the rounds.
You'll have heard it on NPR or possibly from some less useful news source. Today, and today only, you can see a link to it on my APOD gadget to the right.
The Hubble space telescope has imaged, in visible light, a planet orbiting another sun-like star. Yes, we've discovered more than three hundred extrasolar planets to date, but they have always been indirect observations (except one, but it was around a brown dwarf and the "planet" wasn't much smaller than the brown dwarf). We've seen their affects on their parent star, but we haven't seen them directly.
With this Hubble observation, that's changed. A planet about the same mass and radius as Jupiter was imaged as it orbited about 18 billion km around Fomalhaut. 18 billion km is about four times the distance between Neptune and our sun.
That's pretty awesome.
It gets even better.
There's a telescope on the Earth called Gemini. This telescope uses something called "Adaptive Optics" to remove distortions due to the atmosphere. This means that we can get better-than-Hubble images in some instances. Gemini directly imaged, also for the first time ever, a multiplanet solar system in the near-infrared. A third planet in this solar system (dubbed HR 8799) was imaged by another adaptive optics telescope, Keck.
The predictions a few years ago were that we would need large telescopes in space, imaging at the same time, and performing something called interferometry to directly image extrasolar planets.
Now, the above planets were visible to us because they're young. Very young. The planet around Fomalhaut is probably less than 200 million years old, and those around HR8799 are about 60 million years old. The HR8799 planets still hot from their formation, so they're glowing as well as reflecting their stars' light. The FomalhautB planet is so far from its parent star, has thinned the dust around the star just enough that we can see it reflecting starlight.
Regardless, this is wicked cool. We'll be chatting with the Ramans in no time.
Linky to a very excited astronomy blogger.
Wednesday, November 5, 2008
Two good things in 24 hours? Improbable
A visible amount of steam was coming from the tailpipe. White "smoke" from the exhaust means a blown headgasket with coolant getting into a cylinder.
Was this steam evidence of a coolant leak into a cylinder? Well, many new cars have very efficient catalytic converters and one of the products of running exhaust through a catalytic converter is water. That's why you see water dripping from the tailpipe of the person in front of you in a traffic jam. So, this could have just been the expected water condensing more quickly due to the fact that it was ~35F outside.
So, I put a piece of cardboard under the tail pipe, and revved the engine a few times. If there's a leak from the cooling system into the cylinders, this would cause more coolant, possibly some red coolant to come out. All that seemed to come out was water and some carbon, which could have come from the exhaust pipe.
So, I took the truck for a drive. It should overheat if the coolant is being sucked through the cylinders; eventually there wouldn't be any coolant.
I first drove for about a mile or so. No ill effects were obvious.
I got home, shut off the engine and tried to start it again. It started right up. It should have trouble starting if there's a leak for various reasons:
1) No/low compression in one cylinder. This would cause that cylinder not to "fire" correctly. This was not happening.
2) Wet spark plugs. If the spark plugs have water on them, they won't spark correctly. This was not happening.
I checked the oil dipstick for water. There was none.
I need to do some more testing, but it seems like the $15,000 engine suffered no terribly ill effects from being driven more than 10 miles with no coolant (none! Yes, I knew it was stupid, but impatience and frustration often gets the better of my intelligence). I cannot believe this. It's crazy.
Here's the thing that makes it almost believable.
I once almost (by all rights, should have) destroyed another Toyota engine.
I drove my 1989 Toyota pickup from Tucson to Flagstaff to my dad's ranch, ~60 miles east of Flag. Either on the way to my dad's place, over a rough, rocky road, or on the way out from his place, a rock punctured the oil filter. The oil escaped during the trip to Tucson, ~285 miles away. Over the next two weeks or so, I drove the truck around town. There was no oil in my driveway in Tucson; this loss of oil did not occur in Tucson.
Since the truck needed an oil change and I didn't have the time, I made an appointment with a local shop, about two weeks after going to my dad's ranch. On the way to the shop for the oil change, I noticed that the oil pressure was at 0 and the truck hadn't been responding very well. I stopped at the store and put a couple quarts of oil in the engine. When I got to the shop, oil was pouring all over the ground.
This engine had been running on the fumes of synthetic oil for about two weeks. It ran fine afterward.
If my Tacoma really survived this, I believe I have no reason to ever buy a non-Toyota vehicle.
Crazy.
What a wonderful evening
Thursday, October 30, 2008
How do internal combustion engines work?
How do internal combustion engines work? This is not a question posed to me by my son, my friends, or anyone else. I'll explain the purpose of posing this at the end.
An internal combustion engine like what you have in your vehicle works by mixing fuel (gasoline, diesel, etc.) with air, compressing it to very high pressures, (or compressing the fuel to high pressures and then mixing with air in the case of diesel), and then causing the mixture to explode or at least expand quickly, usually via some sort of spark (gasoline, etc.) or other high temperature device (glow plug: diesel). This explosion pushes a piston to move down through the combustion cylinder. That moving piston turns something called a crank-shaft. This crank-shaft is connected to some sort of transmission. That transmission transmits (duh!) the rotational energy from the engine into rotational energy in the wheels via a drive train (which includes a bunch of stuff). There's a flywheel attached to the crank-shaft, which causes the crank-shaft to want to continue turning, even after the piston has reached its lowest point. The crank-shaft is designed such that at the piston's lowest point, it will push the piston back up through the cylinder, starting the internal combustion process anew. This happens over and over and over, causing two to four thousand revolutions of the crank-shaft per minute.
All of these metal parts moving against each other cause tremendous amounts of heat to be generated. The exploding fuel mixture transfers a lot of the waste heat to the engine components. So, there's a system for lubricating and one for cooling an internal combustion engine.
First, the moving parts are immersed in a low viscosity fluid that resists degradation at high temperatures. This is the engine oil. Engine oil is designed as a lubricant that can be heated to high temperatures without changing its properties very much. This lubricant has one main job: to keep the hot metal parts moving smoothly against each other.
The second part to keeping the engine working is the cooling system. There are two parts to this cooling system. First, the engine block is cast (usually in aluminum, steel, or sometimes ceramic) with a network of piping between the outer wall and the cylinders through which a cooling fluid flows. Secondly, a pump pushes that fluid through the block and into a radiator. The radiator has many small pipes which the fluid moves through. This gives the fluid a high surface area, allowing it to quickly cool as relatively cold air passes through the radiator. This works via a mechanism called forced convection (the name "radiator" is a misnomer). That cooled fluid is then pumped back into the engine block and is heated as it moves through the block back to the radiator. The coolant moves around and around, transporting heat from the engine and losing that heat to the surrounding air via the radiator. This effectively and efficiently keeps the engine at a reliable temperature so all of those moving parts can work well.
Here's the thing. The correct, reliable operation of the engine relies on efficient, well-calibrated cooling. That cooling requires coolant. When your radiator develops a hole through which the coolant can escape, the engine can no longer be cooled. When that occurs, a number of nasty things happen:
1) The engine and all of its parts heat up more than they're supposed to.
2) The oil, which is specially formulated to work under specific conditions is no longer working under those conditions, so it starts to degrade.
3) If the oil degrades significantly, it no longer lubricates the moving metal parts, allowing them to scrape against each other.
4) If the oil degrades significantly, it may vaporize, greatly increasing the pressure inside the engine. That pressure increase can be so great that it blows out the gaskets (seals) in the engine block. This is what is meant when you hear someone say they "blew out a head gasket."
5) If the pressure and temperature is a bit higher, the head (the part that the spark plugs and valves and some other components connect to, just above the pistons) can be warped due to the quickly changing size (materials change size when they change temperature) being mitigated in some places by bolts.
6) If the pressure is really high, it can crack the engine block.
I don't know how bad it is yet, but I almost certainly blew out the head gasket on my brand new 11000 mile, 2007 Tacoma. I may have cracked the block, and being the pessimist that I am, I suspect that is the case. Even if I didn't crack the block, the pistons may have scored the cylinders, in which case I might as well have cracked the block (the cylinders in this engine are specially lined and cannot be repaired once they're scored).
Toyota's MSRP for a brand new engine is $15,000. A rebuilt engine runs around $3-4k. If I only blew out the head gasket or warped the head, I can repair it myself for the cost of parts and several weeks to months of working on it in the evenings. If the block is cracked, I need a "new" engine. If the cylinders are scored, I need a "new" engine.
If I need a new engine: I'm toying with the idea of replacing the V6 with an 8-cylinder diesel engine modified to run on biodiesel, veggie oil, or other renewable fuels. But, I need to address some other issues before I can even seriously consider going that route (such as how certain things like stability control and other safety issues are specifically handled and whether they require the gasoline engine to operate correctly). There wouldn't be much difference in cost between a "new" gasoline engine and a "new" diesel engine.
Unfortunately, the truck is sitting in the street by our house because it completely stopped running just as I got home. November 1 is "snow plow" day, meaning we can no longer park on the street.
I cannot/will not start taking apart the engine to assess the damage in the street. I ordered a new radiator from Toyota on Tuesday, but when I went to pick it up today, I discovered they had ordered the one for a manual transmission. The automatic transmission in my truck relies on the radiator to help keep IT at the correct operating temperature. That's another story.
Anyway, I can't move the truck right now because I can't start it without a radiator, which I won't get until Monday. I can't assess the damage because it's not in my garage, and I can't even see how much oil is/was in the cooling system because the hose doesn't reach from the house to the truck (I just bought a new hose this evening while I was out getting the radiator that isn't the radiator), so I can't flush out what's currently in the block to see how bad the damage is.
I HAVE to move the truck before Saturday evening. I happen to have an anchor bolt built into the floor of my garage. I do not know how much stress it can handle, but it's pretty beefy. I plan on attaching one end of a come-a-long to the anchor and the other end to the truck and pulling it up the steep driveway, into the garage. The house happens to be at the top of a hill with a straight shot down the street to a rather cross street. Perhaps you'll see me on the 9 O'clock news this weekend. Don't worry, I'll be careful.
So, I've been rather bummed and not too excited about writing about nuclear energy. My apologies. I'll get back to it this weekend or early next week.
Wednesday, October 22, 2008
Nuclear energy: How it works
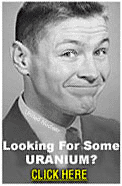
(Kerry loves this little nerd, so asked for it to be included in my post.)
First, I need to say a bit about how atoms (specifically, their nuclei) are put together. This little page has a great primer on nuclear energy in general, and I'll be cribbing from it liberally.
Nuclei are made up of protons and neutrons. These are both relatively massive particles in the atomic world, but the mass of a nucleus is always less than the sum of the individual masses of the protons and neutrons which constitute it. The difference is a measure of the nuclear binding energy which holds the nucleus together. This binding energy can be calculated from the Einstein relationship, which you've seen before. E=mc^2. Only this time, the "m" is a difference in mass between the sum of the individual masses of the protons and neutrons and the actual mass of the nucleus, and E is the energy that holds those neutrons and protons together.
Here is a plot of the binding energy vs nuclide mass or number of nucleons in the atomic nucleus. Nickel 62 is the most tightly bound nuclide. You'll often see it claimed, incorrectly, that Fe56 is the most tightly bound nuclide. This comes from astrophysics where Fe56 is the most common stable nuclide produced in stellar nucleosynthesis processes.

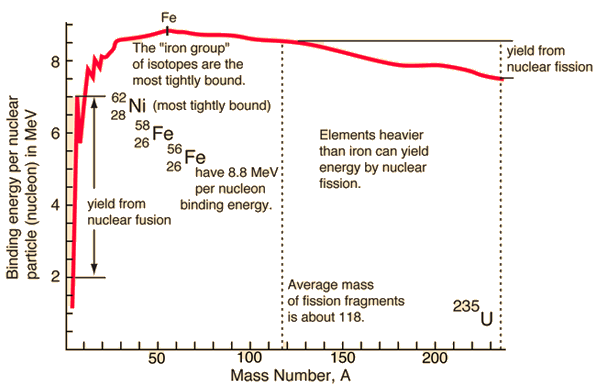

So, as you can see in the plot, you can release the nuclear binding energy by one of two processes. Fission is the process in which you break apart a nucleus of a heavy element (Uranium, for example), creating lighter elements and energy, with some possible other particles released as well. Fusion is the process in which you push two light nuclides together until they form a new element, releasing a bunch of energy. Fusion happens in stars, generating helium and heavier elements from hydrogen. Fission happens in nuclear bombs and nuclear power plants. Fission also happens naturally. We cannot yet get more energy out of a fusion event than we put in, so I will only speak about fission. Interestingly, we can get quite a bit more energy from fusion than from fission, but we need incredible amounts of energy to control the reactions; once we can figure out how to cheaply create the environment at the center of the sun, we can have much, much, much more than enough cheap and "easy" energy... Oh, wait, we have such an environment only 1 AU from us... ;)
Nuclear fission can happen with elements heavier than those in the "iron group", which includes Fe56 and Ni62. The very basic idea is that a slow moving neutron is collided with a massive nuclide (say, Uranium 235). During this collision, Uranium 235 absorbs the nuclide and its energy. Suddenly the nucleus has more energy than the binding energy required to hold it together. This means that there's enough energy to break the nucleus apart. The products are, in one instance, Rb90 and Cs143, a few neutrons, and a bunch of energy. Rb90 and Cs143 are unstable, with half-lives on the order of minutes to seconds; we'll talk about that next time.
Notice that the mass of Rb90+Cs143 is not 235. That missing mass is mostly in the form of energy (about 200 million times as much energy as the slow moving neutron had). There are a few more neutrons as well, but not enough to account for the missing mass. We'll talk about those neutrons in a bit, but first we have to explain "slow moving" neutron.
We need a slow moving neutron because a fast moving neutron won't be captured. In order to control the speed of the neutron, we use something called a neutron moderator. The most common moderator is light water (doesn't have deuterium). That's what is meant by a "light water reactor". There are a number of other moderators in use, and several being researched. These moderators produce what we call "thermal" or "slow moving" neutrons from faster neutrons.
Back to those newly produced neutrons from the neutron+U235 collision. We can use those neutrons to collide with other U235 nuclei, giving us a chain reaction. If we can control this reaction by only allowing one (no more, no less) neutron from each collision to go on to another collision, we can have a sustained but controlled nuclear fission reaction. We control the number of neutrons allowed to participate in collisions by means of the control rods, usually made of boron or cadmium. These elements can absorb many neutrons without themselves becoming unstable. The control rods could get too hot, which would cause them to melt and stop being effective in controlling the chain reaction, causing something like the Three Mile Island accident (like, not identical to).
If we have fewer than one neutron going on to collide with more uranium, the reaction dies and everything stops. No energy generation. If we allow more than one neutron to collide with more uranium, we have an uncontrolled chain reaction with a positive feedback loop, very quickly releasing a lot more energy than we could possibly control in the reactor. This is basically what nuclear bombs do, but it's a lot more complicated, depending on the way things happen.
To maintain the temperature of the control rods, the core, and other components of the reactor, water is used as a coolant. The energy released is so much that the water is quickly converted to steam. This steam is used to drive turbines. The steam-driven turbines generate the electricity, much like coal- or natural gas-fired plants steam-driven turbines.
This has been a very simple and basic description of the process by which nuclear power is used to generate electricity. I'm going to have to add another post or two to discuss the pros and cons and other elements to nuclear energy.
Saturday, October 18, 2008
So am I seeing here that you are an advocate of nuclear energy assuming we were not going to put more effort into solar and wind power?Warning: This is going to be rather opinionated, ranting, and preachy. :)
I'd be interested in hearing more about the nuclear process and the pros/cons of it as a reliable and safe energy source.
The first question is easier. I'm an advocate of reducing pollution, greenhouse gases, and reducing how much of the aeons-old materials we pull from the ground. As was made clear in my first post, basically everything we use comes from the ground. In my opinion, we should take as little mass from the ground as possible. How do we do that?
It's the old mantra: Reduce, Reuse, Recycle. This can be accomplished in many ways, and there are many blogs out there about what specific people do. My bestest friend in the whole wide world (and also my wife) has a bunch of blogs she follows that discuss all different aspects of doing just that.
So, if we reduce the amount of material we're using, we'll very quickly notice that a lot of the energy generation we "needed" is also reduced. We'll notice that the atmospheric pollution we generate is greatly reduced. If we also reduce the amount of energy we directly use (drive less, turn off lights, use more efficient lights and appliances, etc.), we'll notice another, greater drop in pollution.
So, first, I think we should just stop using as much (of energy and stuff) as we use now.
Now, there is some amount of energy we'll need to continue generating to maintain our "standard of living." We can get a lot of that energy from solar, wind, hydrothermal, and hydroelectric. I dare say that we could generate ALL of our energy needs from those four sources. Let me back that up:
There was a math error in the paragraph below. I've corrected it, and had to reduce the fudge factor to a factor of two instead of ten. The point is still the same, it's just not as wildly liberal with how easy it would be to replace all US electricity generation with solar. Corrections are in purple while bad values are in red.
Solar panels are about 12% efficient right now. That means that a cell that covers 0.01 m^2 generates about 1.2 Watts on a clear day. The US uses about 3.2x10^12 watts per year. That means we would need about 2.7*10^12 solar cells that cover 0.01 m^2 each to generate all of our power needs. That's 2.7x10^9 m^2. This is incorrect; the correct value is 2.7*10^10 m^2. That's 27000 km^2. The surface area of the US is 9.2 million km^2 of land. Arizona has about 300,000 km^2. So, let's add 50% of the solar panel surface area to account for the infrastructure. The following statement is a little too liberal due to the math error above. This is a wild guess--we could even double or increase by a factor of 10---it doesn't really matter. Naw, let's use a factor of 10 more, to account for infrastructure, more panels because of the fact that not 100% of the Arizona days are clear, etc. Instead, let's increase by a factor of two to account for infrastructure, etc. So, 54000 km^2 is needed for solar panels to generate 100% of the United State's energy needs. That's about 1/5 of Arizona's land. That's a lot. However, we have a lot of land in the southwest: New Mexico, California, etc. We also have a LOT of roofs in the southwest. We could easily provide more energy than the entire US needs with solar panels alone, at today's efficiencies.
It would be extremely difficult to get all of that energy across the US using the current power grid. Let's add some wind generators in the midwest, some hydrothermal in areas like Yellowstone and Alaska, maybe some wave (I don't like wave much, mostly because I don't know enough about it) on the coasts and Hawaii, etc. We can, with current technology and just a little bit of will, completely stop using coal- and natural-gas fired power plants in a decade or less if we had real leaders in technology and politics, and it wouldn't require the use of ANY nuclear plants.
My next post will directly address nuclear, because I don't think we should necessarily take it off of the table; 27,000 km^2 of solar panels is a LOT of mining for silicon.
Friday, October 17, 2008
Question from my dad
"If we have 1 kilogram of water, what is the maximum amount of energy we could collect from it, assuming Einstein is right and the equation E=mc^2 is correct?"
First, we have to assume some things that are not exactly correct.
1) We could actually "collect" that 'E' above in some fashion.
2) We can turn 100% of the energy into useful work.
So, E=mc^2:
in words:
Kinetic energy is given by the mass of an object times its velocity (we saw this when talking about the small asteroid impacting in Sudan a topic back). Let's assume that we can actually recover 100% of the energy of 1 kilogram of water moving at the speed of light. Ignore the fact that we cannot accelerate a massive particle to the speed of light. The reality of the equation above is that the kinetic energy of 1 kilogram of water (anything, in truth) moving at the speed of light is given by the above equation, not that we can get that much energy from a kilogram of something moving at that speed.
The speed of light is approximately 3x10^8 m/s.
So, the energy involved in 1 kilogram of something moving at the speed of light:
E = 1*(3x10^8)^2 = 9*10^16 Joules (that basically the same as 1x10^17 joules).
Okay. Let's assume we can convert all of that (impossible) kinetic energy into usable energy, say electricity. How long could we power the United States?
According to wikipedia, the US uses 105 exajoules in one year (2005). That's 10^20 joules. So, 1 kilogram of water would provide 1/1000 of the energy used in the US in one year, assuming you could capture and use 100% of the kinetic energy of 1 kilogram moving at the speed of light (ignore that the Lorentz equations require inifinite energy to accelerate anything with mass TO the speed of light).
How much energy do we get from oil? 4*10^19 joules, or about 40% of our total usage. So, if we could capture the energy of 400-500 kg moving at the speed of light and turn 100% of it into energy, we could cease using any oil and continue to power the US at its current consumption rate.
Let's get a little more realistic now.
How much energy can we get from 1 kg of fuel in a nuclear reactor? According to this site, we can get about 1.7x10^11 joules of usable energy in a year. That's about 1 billion times less energy than the US uses. We're currently getting about 8% of our energy from nuclear. That implies that we're using about .08*10^9 = 80,000,000 kg of nuclear fuel today.
However, wikipedia indicates that a large reactor can generate about 10^15 joules from 1 kg of fuel. So, I have an error bar of four orders of magnitude on the energy density of nuclear fuels. That means, if these two sources are at all reliable, we're using something between 8 thousand and 80 million kg of nuclear fuel to provide us with 8% of our energy.
Obviously, this is much better than the mass used in the chemical energy generation (fossil fuel burning). 1 kg of coal will produce about 5.2*10^7 joules of energy. We use about 2.5*10^19 joules of coal-produced energy in a year, so we're using something on the order of 4*10^11 kg of coal a year. We get about 6.2*10^7 joules of energy from one kg of oil. Since we use about 4*10^19 joules of energy (per year) from oil, we're using about 6.5*10^11 kg of oil per year. We use about 2.5*10^19 joules of gasoline per year. We get about 2.6*10^7 joules per kg of gasoline, so we're using about 9.6^11 kg of gasoline per year.
In total, we're using 9.6*10^11 + 4*10^11 + 6.5*10^11 kg = 2*10^12 kg of fossil fuels per year. Compared with up to 8*10^7 kg of nuclear fuel per year.
Even if we decided to go 100% nuclear, with rounding up, we'd be using at most 100 times less material to go fully nuclear. Remember, we're mining both materials out of the ground. Of course, there are issues with making nuclear safe, but there are as many pressing issues with making fossil fuels safe.
[preaching]
The WHO estimates 2.4 million people die each year due to pollution. Let's (unfairly, maybe) lump all of that pollution into that generated by the burning of fossil fuels. Deaths due to nuclear plant operation? About 1000 per year, including all of the disasters and the very slight increases in cancer risk to the public (did you know that coal-fired plants release more radioactive materials than nuclear plants do?).
Here's a nice chart that shows the normalized deaths due to electricity generation:
Fuel | Immediate fatalities 1970-92 | Who? | Normalised to deaths per TWy* electricity |
Coal | 6400 | workers | 342 |
---|---|---|---|
Natural gas | 1200 | workers & public | 85 |
Hydro | 4000 | public | 883 |
Nuclear | 31 | workers | 8 |
Now, add the deaths due to pollution from burning fossil fuels and nuclear power plant-caused deaths are way, way, way below the noise. That's ignoring the increased safety of newer nuclear plants, and that chart doesn't have the deaths due to pollution from burning coal. If you include the deaths due to pollution, that normalized deaths increases to something like 8000 for coal and natural gas.
Imagine we could stop using coal and natural gas and replace them with nuclear, and assume we use the higher number of kg quoted above, just to give us the increase in material we'd need as a proxy for the increase in number of plants we'd need. Assuming a linear increase in the number of normalized deaths with number of plants, we'd have about 800 deaths per TWy for nuclear. Zero for coal+natural gas. That's a net decrease (assuming 8,000 deaths due to coal, natural gas, and pollution) of about 7000 deaths per TWy. The world uses about 16 TW per year. That would mean about 112,000 fewer deaths per year. Considering most of those deaths are due to pollution, we'd also be raising the quality of life of many, many people.
Why isn't the decrease about 2.4 million if all of the deaths due to pollution are due to coal- and natural gas-fired power plants? Because we burn gasoline too, and wood for heating, etc. Also, the above approximations are very poor and linear usually doesn't work well. Given all of that, it's safe to say that a 100% decrease in the use of fossil fuel-fired power plants would make life a lot better for a lot of people, even if we add in the risks due to nuclear fuel.
Of course, if we used less energy, generated a higher % of it from things like solar, created less trash, etc., we'd be even better off.
[/preaching]
Thursday, October 9, 2008
Why is 0 times everything 0?
To answer this question, I first have to give you the field axioms and equation logic:
name | addition | multiplication | ||
associativity | ![]() | ![]() | ||
commutativity | ![]() | ![]() | ||
distributivity | ![]() | ![]() | ||
identity | ![]() | ![]() | ||
inverses | ![]() | ![]() |
Equation logic:
Reflexivity: s = s
Symmetry: if s=t, t=s.
Transitivity: if s=t and t=v, s=v.
Substitution: if s=t and v is a member of the field, s*v = t*v.
Substitution: if s=t and v is a member of the field, s+v = t+v.
There's another rule of logic that's irrelevant to what we're doing here.
These axioms and logic rules define everything you can do with numbers in the various fields (complex numbers, real numbers and rational numbers, for example. The set of integers is not a field because you cannot mathematically invert any given integer and get an integer back.
Okay, let's use the above axioms and equation logic to prove that, for any number A that is part of a field, A*0=0.
1: 0=0 (reflexivity)
2: 0+0=0 (additive identity)
3: A*(0+0) = A*0 (logical substitution; multiplication is well-defined).
4: A*0 + A*0 = A*0 (multiplicative distribution)
5: A*0 + A*0 + -A*0 = A*0 + -A*0 (logical substitution; addition is well-defined)
6: A*0 + (A + -A)*0 = 0*(A + -A) (multiplicative distribution)
7: A*0 + 0*0 = 0*0 (additive inverse)
8: let 0*0 = b. (simplification, and therefore:)
9: A*0 + b = b
10: A*0 + b + -b = b + -b (logical substitution; addition is well-defined)
11: A*0 + 0 = 0 (additive inverse)
12: A*0 = 0 (additive identity)
13: QED (pompousness)
There you have it. For any number A in a field that obeys the above axioms and rules of logic, A*0 = 0.
Tuesday, October 7, 2008
And we have impact.
The Sudan atmospheric impact's energy release was between 1.1 and 2.1 kilotons of TNT. That's about 1012 calories or 4.184 terajoules. Little Boy released between 13 and 18 kilotons of TNT equivalent energy. Tunguska, another atmospheric asteroid explosion released about 5 to 30 megatons of TNT equivalent energy. These little rocks are moving so fast that they could really do some damage...
[rant]If you think NASA's budget is too high, consider the above information carefully. We can now detect rocks just a few meters in diameter. Such small rocks release 1/10 of the energy of the atomic bomb that destroyed Hiroshima. A rock just a little more massive, faster or bigger is a pretty scary proposition. An asteroid a lot bigger or more massive is quite nasty and we need to better understand our solar system so we can better understand our place in it.
We can, with some basic assumptions, work out the density of this asteroid, knowing nothing more than the amount of energy released, the size, and the escape velocity of Earth. I assume this information is somewhere out there, but let's just do this for fun. ;)
We all know that the kinetic energy of a body is its velocity squared times its mass. An object impacting the earth from space is moving close to the earth's escape velocity.
E=mv^2.
E = 4.184x10^12 J
v=11.86 km/s = 11860 m/s
(the actual velocity was 12.8 km/s, so let's use that instead of the escape velocity of the Earth)
m = E/v^2
m = (4.184x10^12 kg m^2/s^2)/(12800m/s)^2
= 4.184x10^12 kg m^2/s^2)/(163840000 m^2/s^2)
= 25,537 kg
Assume a spherical rock 3 m in diameter. Its volume is 4/3 * Pi * r^3 = 4/3 * Pi * 1.5^3 = about 14 m^3.
The density of an object is mass divided by volume. 25537/14 =1824 kg/m^3. That's the density of a rubble pile.
This asteroid was not made of nickel/iron. Nor was it solid rock; this was rock with lots of pore space (gaps between the rocks).
Let's go back to my little rant about more massive rocks. Let's assume this rock, with the same density, was ten times larger in diameter (not too unrealistic).
Its volume would be: 14000 m^3. With a density of 1800 kg/m^3, its mass would be 25,200,000 kg. If it were moving at the same velocity of 12800 m/s, the total energy released would be:
E=mv^2
E = 25,200,000 kg * (12800 m/s)^2
E = 25,200,000 kg * (163840000 m^2/s^2)
E = 4.12 * 10^15 joules.
That's about a megaton of TNT. That's 100 times as powerful as the bomb that destroyed Hiroshima.
Monday, October 6, 2008
Not a question...
This is an email forwarded to me from...well, someone else. ;)
NEO News (10/06/08) Bolide impact predicted tonight!
This is a first: a very small asteroid (or rock) has been discovered that is on course for an impact tonight in Sudan. This information is from various reports to posted to MPML (the Minor Planet Mailing List at groups.yahoo.com/group/mpml/). The impactor is only about 2 m across and will break up in the atmosphere, with no risk to those on the ground. (If something this size hit in the daytime, it would probably not be noticed, but at night it should put on quite show).
Alan Harris writes that this object, with the survey-assigned designation 8TA9D69, was discovered by the University of Arizona Mt. Lemmon survey and will almost certainly, tonight, become the first impacting bolide discovered before entry into the Earth's atmosphere. Steve Chesley (JPL) reports that atmospheric entry will occur on 2008 Oct 07 0246 UTC over northern Sudan.
Andrea Milani of the University of Pisa wrote the following: Today the object with the provisional designation 8TA9D69 was submitted to impact monitoring by using the normal software of the NEODyS system, by using the observations as reported by the MPC on the NEO Confirmation Page. Based on 26 optical observations from 2008/10/06.278 to 2008/10/06, the probability of impact is between 99.8% and 100%; in practice the impact can be considered sure and is for tonight. Our computation has already been confirmed independently by others, including the JPL NEO Program Office (with which we consult in all relevant cases of possible impact). The effect of this atmospheric impact will be the release, in either a single shot or maybe a sequence of explosions, of about 1 kiloton of energy. This means that the damage on the ground is expected to be zero. The location of these explosions is not easy to predict due to the atmospheric braking effects. The only concern is that they might be interpreted as something else, that is man-made explosions. Thus in this case, the earlier the public worldwide is aware that this is a natural phenomenon, which involves no risk, the better.
This is the first time an asteroid impact has been predicted, and it reflects the increasing capability of the Spaceguard Survey. There was one previous false alarm when, for a few hours around Christmas 2004, it appeared that an impact by a 30-m asteroid was possible, but this was ruled out by additional observations. The current case, however, seems much more solid.
Two meters! My size (in one dimension anyway)! That's the size of the object detected, tracked, and confirmed by independent observers, with 98%+ probability, to hit the Earth's atmosphere tonight (I believe it'll be Sudan's night). If you don't think this is awesome, wicked cool, and other sundry adjectives, I don't know what to tell you. :)
Friday, October 3, 2008
How is fuel made?
Oh, boy.
First, the crude oil is extracted from the ground. That's a different story...
Linky
The crude oil is brought to a refinery, where it is pumped through a high temperature steam boiler. This boiler heats the crude oil to about 600 to 700 degrees celsius. This superheated crude oil is pumped into a distillation tower.
The distillation tower has several levels of progressively lower temperatures, with the highest temperature at the bottom and the lowest at the top. Most of the components of the crude oil will vaporize at or below 600 C. Because of the temperature profile of the refining tower, certain chemicals will be condensed at certain heights. This difference in condensation temperature allows the separation of chemicals as their gaseous forms rise through the tower.
The stuff that won't vaporize at below 700 C or so is called the residual crude---tar, coke, some waxes, etc. These are solids or very refractory liquids that are often used in making other things.
At about 370 to 600 degrees C, heavy oil condenses. This is used as an industrial fuel or used to make other products.
At about 300 to 370 degrees C, lubricating oil (stuff used to lubricate your car engine), grease, etc.) condenses.
At about 250 to 350 degrees C, diesel fuel, heating oil, etc. condense. These are sometimes used to make other products.
At about 175 to 325 degrees C, kerosene condenses. Kerosene is used in jet fuel, tractor fuel, lantern oil, etc. Often used to make other products, too.
At about 40 to 205 degrees C, gasoline condenses. This is the fuel most of us use in our cars.
At about 60 to 100 degrees C, naptha condenses. Naptha is used to make high octane gasoline, as an industrial solvent, etc.
At about 40 degrees C, petroleum gas condenses. This is used to make LPG, to make plastics, etc.
Sunday, September 28, 2008
How are hydrogen and oxygen created?
That was it. The universe expanded and cooled too quickly for any more elements to form.
It took around 200 million years for the first stars to form. Those first stars (population III or first-generation) fused hydrogen and helium to create the 88 heavier elements, which were released when the stars died and exploded. Oxygen is one of those elements.
So, there you have it; we are recycled star dust.
Garion's response: "Cooool!"
Thursday, September 25, 2008
Why do leaves change color?
Oh, good, an easier one. :)
Green plants use a chemical called chlorophyll to absorb sunlight, which is needed to metabolize carbon dioxide and water into the sugars the plants need to grow. The carbon dioxide is absorbed from the air (through the leaves, via a process called respiration), and the water is absorbed through the ground.
When fall comes, the days get shorter (less sunlight), which signals the plants to stop producing so much chlorophyll. The plants need to produce less chlorophyll because there's less sunlight and because the winter is generally drier than the rest of the year. So, because there's less sunlight and less water, the plants basically go into hibernation (wrong word, of course) until the days become longer, warmer, and wetter.
Because the plants are not producing chlorophyll, the green color of the leaves fades and the less dominant colors come out. Those colors were there all along, of course, but they were overwhelmed by the green from the chlorophyll.
How do people walk? Part II
The biological answer.
(I will only discuss the muscle contraction part. I'm not a biologist, and there is a HUGE body of research on the biomechanics of walking; I couldn't do it justice.)
When you decide to pick up your foot and move it forward, the brain stimulates a neuron called an alpha motor neuron. This in turn sends a signal down its axon to stimulate the release of calcium (hey, that's from rocks!), which binds to troponin. This chemical reaction causes protiens on the actin (tropomyosin) to change their configuration slightly, exposing a site on the actin to which the myosin head binds.
So, now the muscle is in a configuration such that a thick filament is bound through the myosin heads to a thin filament. The thin filaments are more dynamic than the thick filaments and with the application of a little energy, the thin filament will be "walked" back toward the thick filament. The energy comes from hydrolysis of ATP, which basically transports chemical energy throughout the body.
All of this happens many, many times just to cause your muscles to contract a little bit. To walk, you do this (with many different muscles) over and over and over again.
So, here's a nice little animation of muscle contraction.
Tuesday, September 23, 2008
How do people walk?
(K's Answer: "Put one foot in front of the other.")
Okay. There are two parts to this question. 1) physics 2) biology
I'm only going to answer the first part (and really only one very small but important part of that) tonight. I'll try to get to the biology part later in the week.
Basic physics of walking:
1) You all know that we need friction to get anywhere. The ground exerts a frictional force against our forward foot, causing it to stay put while we swing our rear foot around the pivot (hips), advancing our center of gravity; this movement is very much like a pendulum, which I'll get to later.
We can actually write a little expression for the (approximate) minimum coefficient of friction required [linky]:
m = v /[6gL / p2 - v2]1/2
Where m is the coefficient of friction, v is your speed, g is the gravitational acceleration Earth exerts on you (9.8 m/s2) and L is the length of your legs. So, for someone like me who has, ~1 meter long legs, moving at ~2 m/s (4.4 mph), the minimum coefficient of friction required is about 1.4.
That's a unitless number that basically tells you that the frictional force stopping my foot from moving must be 1.4 times the force gravity exerts on me. This is not strictly correct, but I don't have the space to go into it; suffice to say that the amount of force you apply in the opposite direction of your movement must be no larger than the frictional forces. The coefficient of (static) friction only makes sense in this context: when the frictional and applied forces are perfectly balanced, just before your foot slips.
As I try to go faster, I exert greater forces that attempt to cause my feet to slip, and I need a greater coefficient of friction (or more appropriately, the frictional forces need to be higher to keep my feet from slipping, which requires a greater coefficient of friction). If I try to walk on ice, I cannot move very fast because the coefficient of friction is so low that the forces required to move my feet faster tend to easily overwhelm the frictional forces attempting to keep my foot from moving and I slip (and then I usually fall).
I'll talk about the pendulum-like behavior of our bodies next time, when I also get to the biology of walking.
Thursday, September 18, 2008
How do rocks create everything? or do they?
"How do rocks create everything? or do they? I mean everything not animal or person."
He is not going to make this little experiment easy!
First, I'm going to assume he means on the Earth. Bad assumption, I know, but I've got to start somewhere... ;)
First, let's define a rock. Wikipedia has a decent definition. A rock is a naturally occurring aggregate of minerals or mineraloids. A mineraloid is a chemical substance that doesn't actually exhibit a crystalline structure, but perhaps would under different circumstances. Think opal or obsidian; amorphous structures that, in large aggregates, are often considered rocks.
Okay, so a rock is basically a (naturally occurring) collection of minerals. For now, I'm not going to go into how rocks themselves are formed. Let's explore how rocks (minerals and aggregates of minerals) "make everything."
- Plants: Plants require a certain subset of minerals (actually, a certain subset of mineral-forming elements) to grow. There are about 13 elements that form minerals of various kinds that the typical plant can use. This site has a good description of which mineral-forming elements plants use (by absorbing the water into which the minerals have dissolved). I'm going to list the mineral-forming elements and then move on (there's just not enough room to discuss each element--follow the link above if you're interested). All of the minerals come from the soil, which comes from rocks.
- Nitrogen (N)
- Phosphorus (P)
- Potassium (K)
- Calcium (Ca)
- Magnesium (Mg)
- Sulfur (S)
- Boron (B)
- Copper (Cu)
- Iron (Fe)
- Chloride (Cl)
- Manganese (Mn)
- Molybdenum (Mo)
- Zinc (Zn)
- Building materials: Nearly every kind of building material you can think of utilizes rocks in one form or another.
- Portland cement is the most commonly used cement in the industrialized world, and its basic components are often used unprocessed as a cheap cement. Portland cement is made up of calcium oxide, silicon oxide, aluminum oxide, ferric oxide, and magnesium oxide is specific concentrations. The minerals are usually quarried from local rocks.
- Drywall (wallboard, greenboard, etc.) has an inner core made up of gypsum (CaSO4 * 1/2 H2O), calcium sulfate.
- Shingles, roofing tiles, etc. Many materials used to keep the interior of a home or building are made from minerals of one kind or another. Much of Switzerland has houses with slate roofs, the common shingles you see on most houses (in the west anyway; I don't know anything about the east) are made from tar, fiberglass, and minerals of the desired color. The "mexican" roofing tiles are made from clays and other minerals and then baked.
- Bricks, cinder blocks, flooring tiles, etc. are generally made of either clays or ceramics, both of which are formed from minerals, which (of course) come from rocks. And don't forget the grout which is a fine-grained cement which often has sand and sometimes very fine gravel mixed in.
- Sinks, bathtubs, etc. are often made of ceramics or other mineral-bearing materials.
- Counters and tabletops can be tiled (with grout) or made of whole slabs of rock.
- Windows are made of glass (there are many different kinds of glass, many of which contain silica), which is an amorphous solid, rather than a crystalline solid. However, the minerals that are melted and quenched to make the glass almost always come from rocks.
- Lightbulbs are also made with glass and often metal.
- Wood, paper, etc. are made from plants that require minerals to grow.
- Nearly all metals are smelted from mineral ores. Aluminum and brass fixtures, iron pipes, copper pipes, door knobs, electrical wires, nails, screws, bolts, etc. all come from ores (or recycled metals, which originally came from ore).
- I'm sure I've missed a million things.
- Personal Hygene/makeup: Lipstics, makeups, toothpastes (hydrated silica--opal, calcium carbonate--chalk, titanium dioxide) , etcetera, etcetera, etcetera all often have minerals as part of their basic composition, often for coloring or sparklies (mica) in the makeup.
- Dishes, flatware, silverware, cookware, etc: China, ceramic, glass, metal, clay, etc. are all made of minerals or smelted from minerals or somehow derived from rocks.
- Magazines: That shiny paper used in magazines? it's made by coating paper in clay.
- Electronics: The metals in all electronics are originally derived from ores (rocks). The glasses used in computer displays, etc. are derived from minerals. The silicon used in the chips is derived from rocks. Solar panels, cameras, radios, etc., etc., etc., all contain silicon and metals, which are both derived from rocks.
- Pigments: Many inks and pigments are made by crushing certain minerals and mixing them into a suspension. Those that don't come from minerals come from plants (which use minerals to grow...blah, blah, blah).
- Everything else, practically. About the only thing that I can think of that doesn't come from minerals directly or through one generation or so is petroleum (and therefore plastics). Petroleum (etymology: "rock oil") is plant and animal matter that's been heavily altered by very high pressures and temperatures for very long times. At some point, one has to question if it hasn't changed so much that it's no longer even close to being similar to its original source. Of course, it's pumped out of the ground where it is intimately mixed with rocks... Water, atmosphere, and a few other things are also examples of substances that are removed from rocks by multiple-generations (although many parts of the atmosphere are derived--sometimes directly--from rocks). It really is true that if it can't be grown or hunted, it must be mined [linky]
Okay, I got lazy and didn't want to dig up more examples of how "rocks make everything." I also got lazy and didn't want to add more links. I'll probably come back here and insert reference links when I have a little more time.
Introduction
--G
--M